Every day, scientists, engineers and technicians at Fermilab push the boundaries of knowledge in fields like particle physics, accelerator technology, quantum information science and astrophysics. Read about 10 ways the laboratory advanced science and technology in 2024. Additionally, a video highlighting the laboratory’s accomplishments may be viewed here.
1. Achieved important progress with DUNE
Fermilab is the host laboratory for the Deep Underground Neutrino Experiment. This international collaboration will explore the mysteries of elusive particles called neutrinos. More than 1,400 scientists from over 35 countries and CERN are part of the collaboration that is seeking to answer some of the biggest questions around our understanding of the universe. DUNE will be installed in the Long-Baseline Neutrino Facility, currently under construction in Lead, South Dakota at the Sanford Underground Research Facility, and at Fermilab in Batavia, Illinois. Crews completed excavation of DUNE’s caverns in February, removing close to 800,000 tons of rock from a former mine in South Dakota for the future subterranean home of the experiment’s far detector. A ribbon-cutting event was held in August with officials from around the globe commemorating this historic milestone. In September, a test for lowering steel beams was successfully completed in preparation for the next phase of the project.
Meanwhile, in Illinois, the Fermilab accelerator complex achieved a critical milestone towards high intensity running for DUNE, reaching 1 MW beam intensity from its Main Injector. Additionally, workers prepared the eight acres at Fermilab where the future DUNE near site will be built. And a prototype for the near detector — the 2×2 demonstrator — saw its first accelerator-made neutrinos. Across the pond at CERN in Geneva, Switzerland, prototypes for the far detector — ProtoDUNE — were filled with liquid argon to ready them for operation.
This year, Jim Kerby was appointed the new LBNF/DUNE-US project director. Kerby brings over 30 years of engineering and technical management experience to the table. He will be responsible for managing all aspects of the project in the U.S. as Fermilab leads the execution of the largest international DOE project ever hosted on U.S. soil.
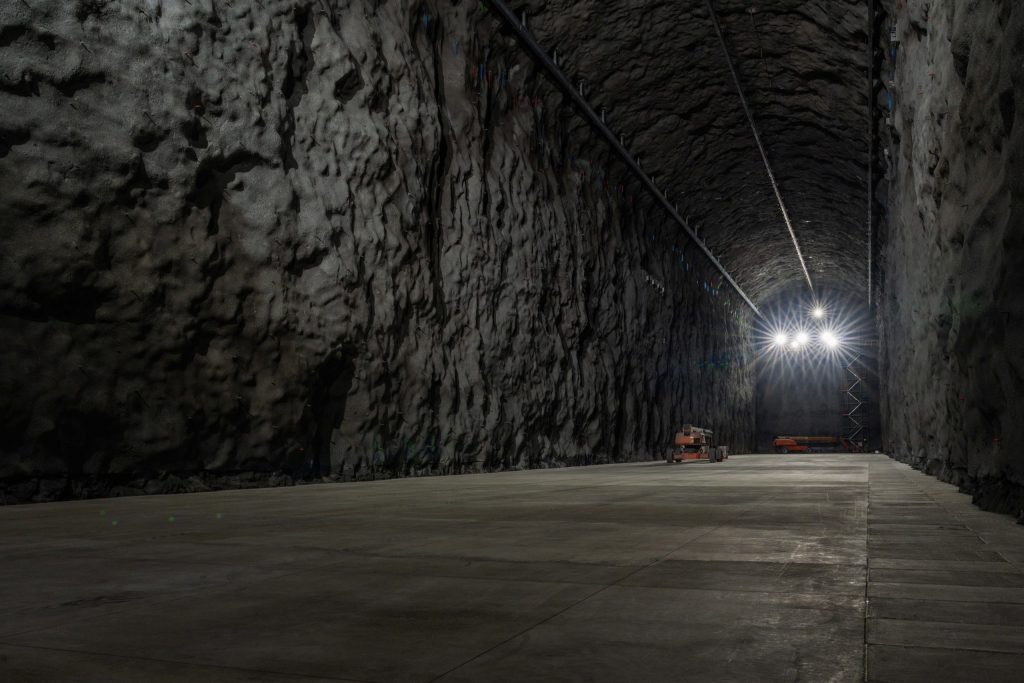
2. Made major advancements with the Proton Improvement Plan-II project
Proton Improvement Plan-II is providing a major upgrade to the Fermilab particle accelerator complex, including a state-of-the-art superconducting radio frequency linear accelerator. The PIP-II project started off the year by welcoming a new director, Pantaleo Raimondi, a world-renowned physicist with extensive experience in accelerator physics and project management at labs around the world.
The PIP-II team also made progress with one of the five types of cryomodules that will make up the linear accelerator. Fermilab successfully shipped a prototype high-beta 650-megahertz cryomodule — the largest needed for the PIP-II linac — to the United Kingdom and back again. This was an important step in testing the cryomodule transportation system and a final test before shipping the first actual cryomodule built in the U.K. to the United States.
PIP-II is the first particle accelerator in the U.S. to be built with significant contributions from international partners. Institutions in France, India, Italy, Poland and the U.K. are contributing technologies, instrumentation and expertise to build the accelerator. Early in 2024, India’s Department of Atomic Energy informed the U.S. Department of Energy that India is officially moving from the research and development phase to the construction phase for its contributions to the PIP-II project. Pieces of India’s largest in-kind contribution to PIP-II, the cryogenic plant, are scheduled to arrive at Fermilab in the next month after a two-month journey over sea and land. In addition, PIP-II partners at UK Research and Innovation received the first production HB650 cavity, which was tested and met specifications. And at INFN, the National Institute for Nuclear Physics in Italy, PIP-II partners are close to awarding the contract to produce all low-beta 650-megahertz cryomodule cavities.
In November, the project completed the Early Conventional Facilities subproject, marking the subproject’s readiness for the final stage of approval, known as CD-4, planned for January 2025.
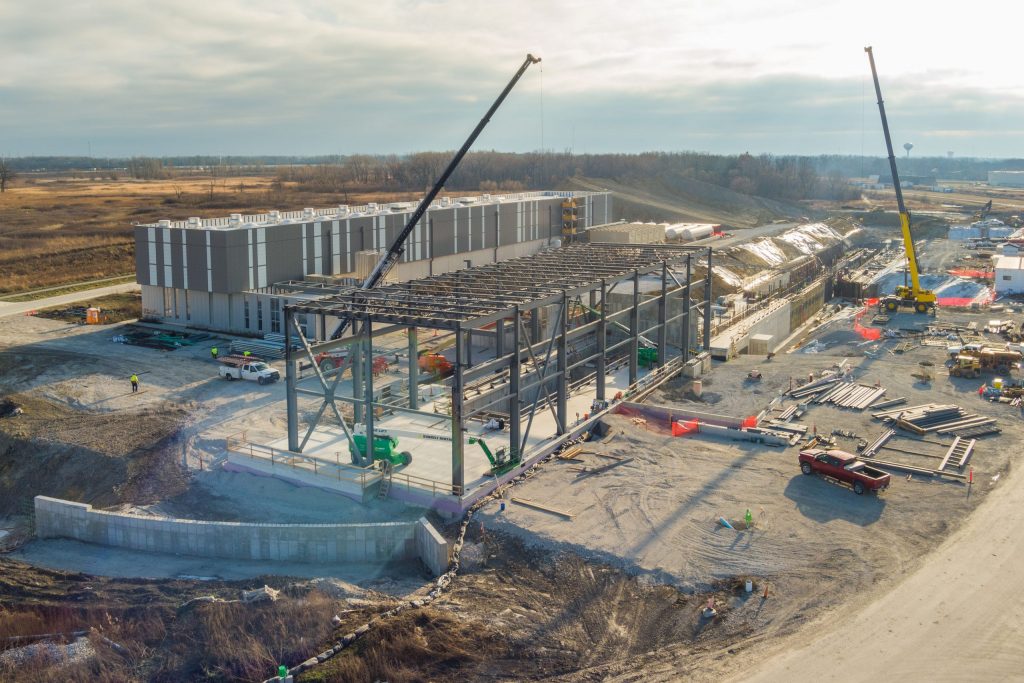
3. Continued our involvement in the CMS experiment at CERN
For decades, Fermilab has been the host institution for U.S. CMS. The CMS experiment at CERN records data from high-energy particle collisions produced by the Large Hadron Collider, the world’s biggest particle accelerator. Earlier this year, Fermilab scientists working on CMS helped create a tool that expands the search for new particles at the LHC. The search could either uncover physics beyond the Standard Model or set the most stringent limits in the search for a class of theoretical particles called long-lived particles. In September, the CMS collaboration announced a new mass measurement of the W boson, one of nature’s force-carrying particles, that is consistent with predictions. This new measurement, which followed the 2022 measurement by the Collider Detector at Fermilab experiment that differed from the Standard Model prediction, is the most elaborate investigation of the W boson’s mass to date and took nearly a decade of analysis.
The Department of Energy also approved the start of full production for the $200 million DOE-funded contributions to the upgrade of the CMS experiment. With the high-luminosity upgrade to the Large Hadron Collider planned for 2029, CMS collaborators need to upgrade the detector to keep up with the forthcoming more-intense particle beams.
Fermilab connections continue to be strong at the highest levels of the CMS collaboration. Patty McBride, a Fermilab distinguished scientist, completed her two-year term as the CMS spokesperson in September. She passed the torch to a new management team that includes Fermilab senior scientist Anadi Canepa, now a deputy spokesperson for CMS until 2026.
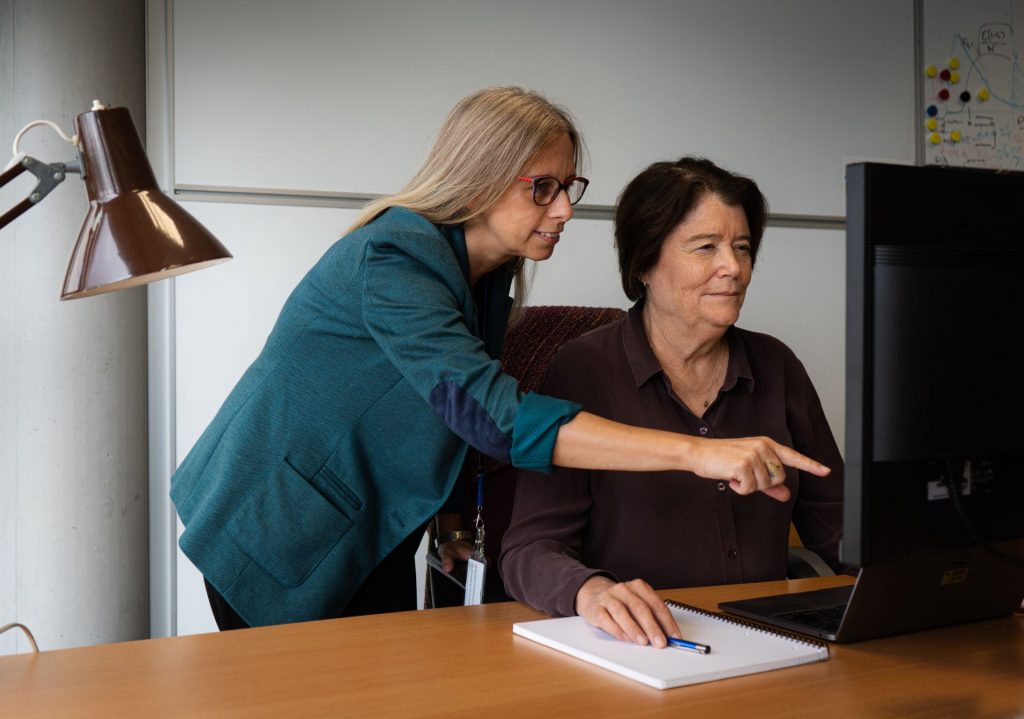
4. Detected first neutrinos at the Short-Baseline Neutrino Detector
The international Short-Baseline Neutrino Program at Fermilab is devoted to examining the properties of neutrinos and the nature of neutrino oscillations in more detail than ever before. The Short-Baseline Near Detector is the near detector for the SBN Program while ICARUS, which started collecting data in 2021, is the far detector. A third detector called MicroBooNE finished recording particle interactions with the same neutrino beamline that same year.
After nearly a decade of planning, prototyping and constructing the near detector, SBND made major progress in 2024. In February, SBND was filled with liquid argon, which it uses to see tracks left by charged particles. A few months later, the detector saw its first neutrino interactions. But it’s only the beginning for SBND: the collaboration will operate the detector, analyzing many millions of neutrino interactions, for the next several years. SBND will see more neutrinos than any other detector of its kind, and the large data sample will allow researchers to study neutrino interactions with unprecedented precision, helping to inform future experiments that will also use liquid argon to detect neutrinos, including DUNE.
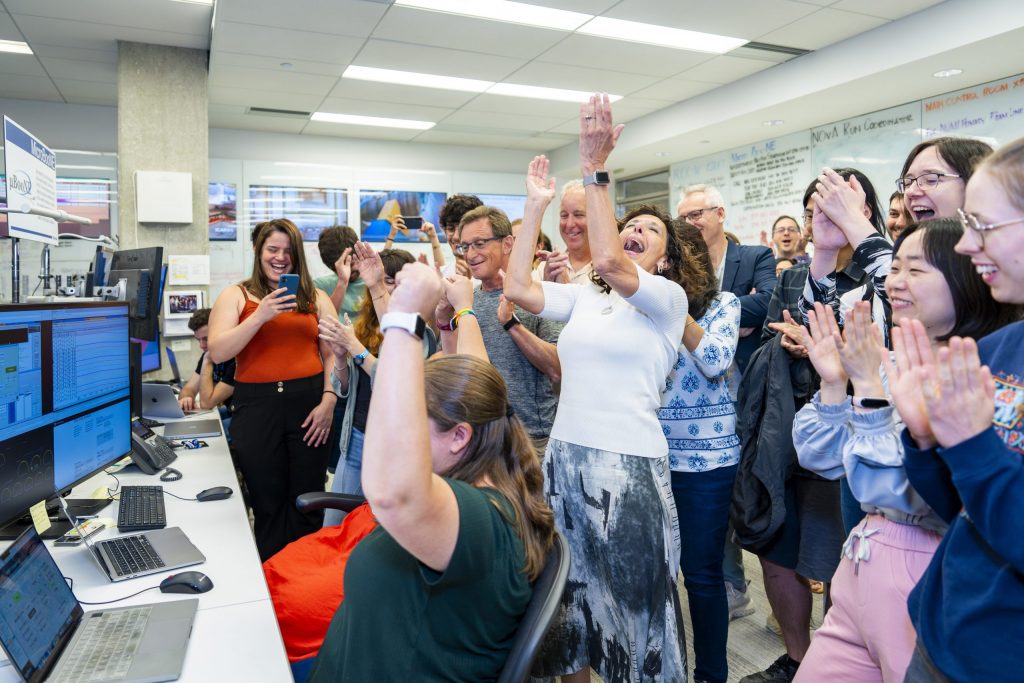
5. Moved massive magnets
In February, crews very carefully moved a superconducting solenoid magnet 1.5 miles across the Fermilab campus. The 65,700-pound magnet was built for the Mu2e experiment, which is looking for evidence that a muon can transform into an electron. If observed, this muon-to-electron conversion would point to new physics. The team moved the first Mu2e magnet in December 2023. Once assembled into the Mu2e detector, the magnets will create a low-energy muon beam that will be directed at an aluminum target. The magnets will also provide a constant magnetic field in the detector region that allows scientists to accurately determine the momentum of the resulting electrons.
Over the summer, a different kind of magnet weighing over 100,000 pounds was moved from the University of Illinois Chicago to Fermilab. The repurposed superconducting magnet will be used in a future experiment.
In late fall, Fermilab shipped its second quadrupole magnet cryoassembly to CERN. This magnet is part of Fermilab’s contribution to the high luminosity upgrade of the Large Hadron Collider. It uses advanced niobium-tin (Nb3Sn) magnets to strongly focus the proton beams and increase the number of collisions. Fermilab innovations were crucial to making these high-field magnets possible.
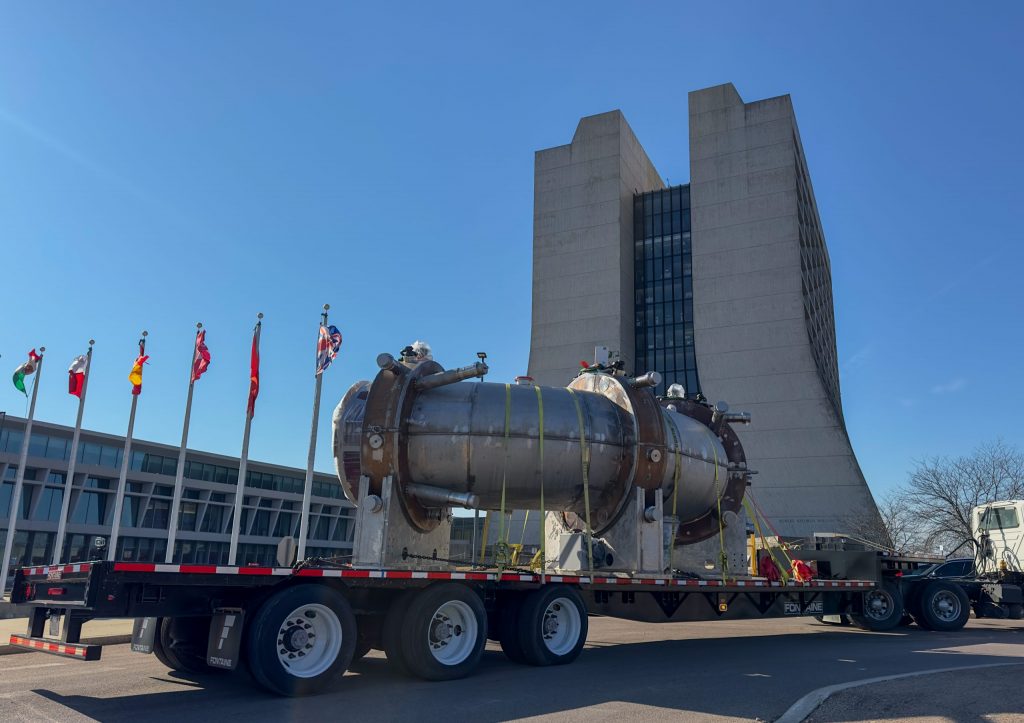
6. Strengthened our leadership in quantum information science
Fermilab is the proud host of the Superconducting Quantum Materials and Systems Center, one of the five DOE National Quantum Information Science Research Centers. The SQMS Center brings together more than 30 partner institutions representing national labs, industry and academia, all dedicated to advancing critical quantum technologies with a focus on superconducting quantum systems.
During 2024, SQMS scientists and engineers achieved reproducible improvements in superconducting transmon qubit lifetimes with record values in excess of 1 millisecond. The results were achieved through innovative materials and design techniques that eliminated major loss sources in the devices. SQMS has also advanced quantum computing platforms based on high-coherence superconducting cavities.
Over the summer the Department of Energy approved IBM as a new partner in SQMS. This collaboration intends to leverage the strengths of these two organizations to address key hurdles in quantum computing, communication and large-scale deployment of superconducting quantum platforms.
This year, SQMS led the NQISRC’s executive council, coordinating joint activities across the five centers, which have strengthened the national quantum information science ecosystem, achieving scientific and technological breakthroughs as well as training the next-generation quantum workforce.
Quantum technology can also be used to probe the fundamental theory of quantum mechanics. Fermilab theorists and experimentalists used qubits to constrain alternatives to the standard laws of quantum mechanics in which systems evolve linearly in time.
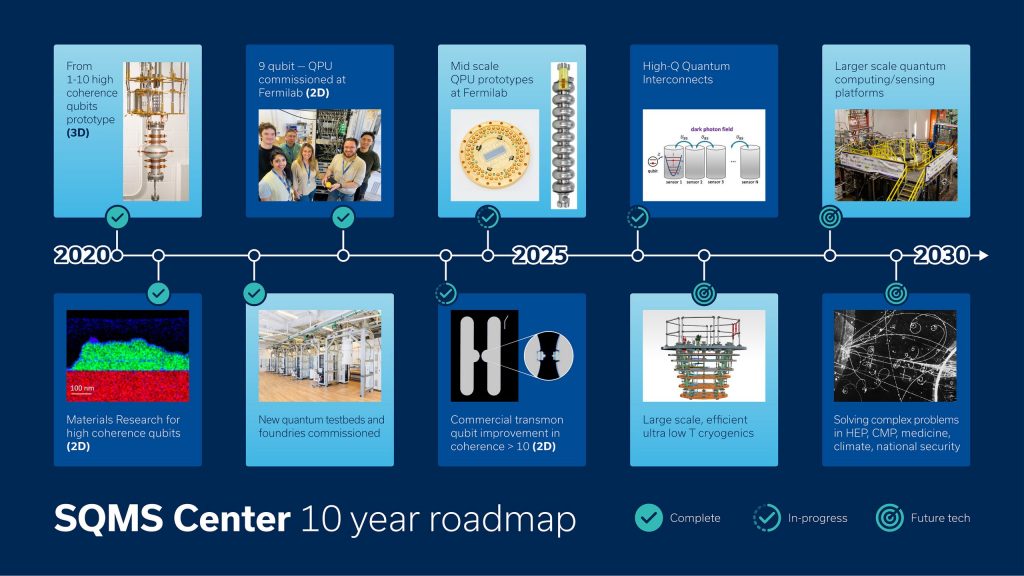
7. Got very QUIET
In June, a new quantum sensor and computing research center named the Quantum Underground Instrumentation Experimental Testbed became operational. QUIET sits one hundred meters underground at Fermilab in an area that previously housed a neutrino experiment. Its companion surface lab, LOUD, had been operating for over a year prior to QUIET’s opening. Together, QUIET and LOUD enable controlled experiments that use quantum sensors to directly compare an environment that is significantly shielded from cosmic rays and other energy effects with the environment on the earth’s surface.
In October, superconducting qubits were successfully deployed at QUIET for the first time, marking the transition from infrastructure development to unique scientific studies at the lab. Scientists are using QUIET to understand how these superconducting qubits are impacted by cosmic rays and other high-energy particles. This knowledge could help researchers construct new types of qubits that could be shielded from interference or design ones that are insensitive to it. In addition, QUIET can contribute to a range of applications that require ultra-sensitivity to their environment, including dark matter detection. QUIET and LOUD are funded by the Quantum Science Center, of which Fermilab is a primary founding member.
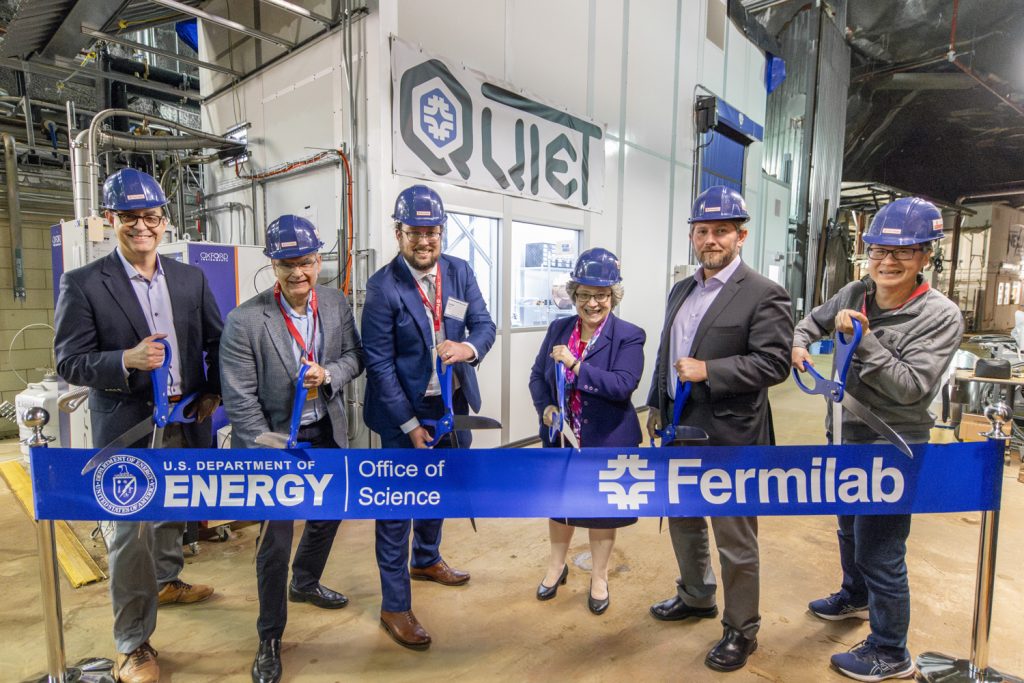
8. Learned more about dark energy and our universe
We’re not just about particle physics! Astrophysics is an important piece of Fermilab’s portfolio. In 2024, Fermilab researchers continued to shed light on some of the greatest mysteries in the cosmos — such as dark energy, the enigmatic entity that makes up about 70% of our universe. Fermilab scientists lead the Dark Energy Survey, an international collaboration of over 400 astrophysicists, astronomers and cosmologists, which shared two results in 2024. In January, they announced the strongest constraints on the expansion of the universe ever obtained with the DES supernova survey. A month later, the collaboration released a new measurement of cosmic distances that supports the standard model of the accelerated expansion of the universe.
This year, researchers released the first results from the Dark Energy Spectroscopic Instrument, which is gathering light from some 30 million galaxies at a telescope at Kitt Peak National Observatory. The DESI collaboration used the first year of data to make the most extensive 3D map of our universe and world-leading measurements of dark energy. They also charted how nearly 6 million galaxies cluster across 11 billion years of cosmic history, lining up with predictions of Einstein’s theory of general relativity. Fermilab contributed key elements to DESI, including the online databases for data acquisition, software to control the robotic positioners, the corrector barrel, hexapod and cage.
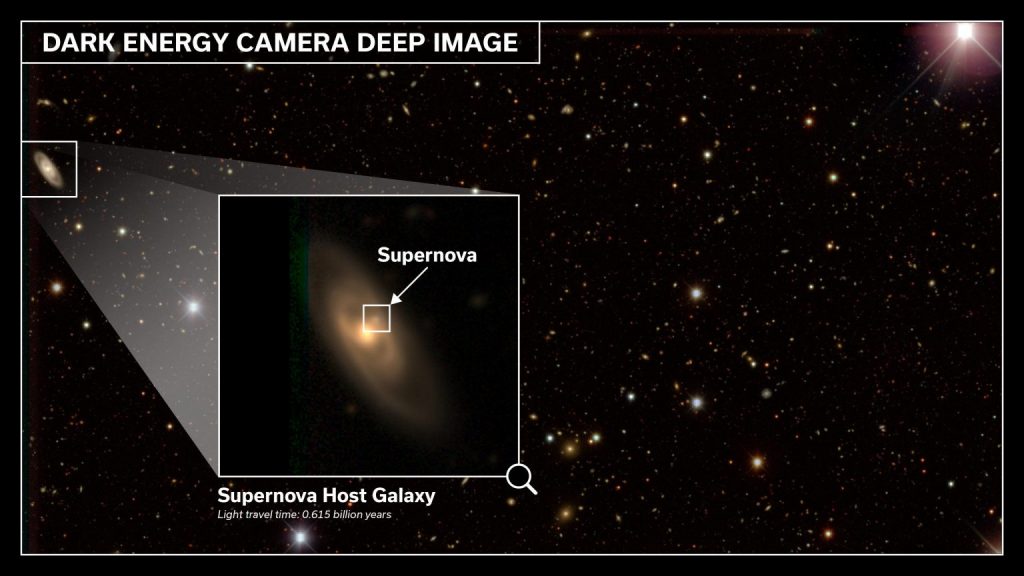
9. Advanced emerging technologies to benefit physics and beyond
Fermilab’s contributions to research and technology development reach well beyond physics. In collaboration with 3M, Fermilab scientists successfully demonstrated that an electron beam can destroy PFAS, a suite of useful chemicals that don’t easily break down and accumulate in the environment and human body. Fermilab researchers are also building a prototype electron beam accelerator to make X-rays for sterilizing medical equipment — a potentially game-changing development for the growing medical equipment sterilization industry, which is looking for alternatives to current technologies that use substances that can present safety issues.
This year, Fermilab researchers also received funding from the Department of Energy as part of its Accelerate Innovations program to develop three different emerging technologies: superconducting nanowire single-photon detectors, 3D integrated sensing solutions, and compact superconducting radio frequency electron-beam accelerator technology. An additional federal grant enabled a collaborative project between Fermilab and California-based RadiaBeam Technologies. Fermilab engineers used their expertise in cryomodule design and conduction cooling to help RadiaBeam design and assemble a conduction-cooled cryomodule and break into the superconducting industrial accelerator market.
In another quantum experiment, Fermilab scientists demonstrated the ability to use specialized quantum techniques to stimulate the creation of photons, increase sensitivity and minimize noise. This research can significantly enhance the ability to detect faint signals such as those emitted from dark matter.
Lastly, this month, Fermilab engineers announced they are ready to bring to market a new companion to the Quantum Instrumentation Control Kit, an open-source control and readout system supported by the Quantum Science Center. The new product, QICK box, builds on QICK’s ability to enable researchers to improve quantum system performance by manipulating signals in ways that optimize their ability to read information stored in quantum bits. In September, the team also rolled out QICK version 2.0, which features updated software and firmware.
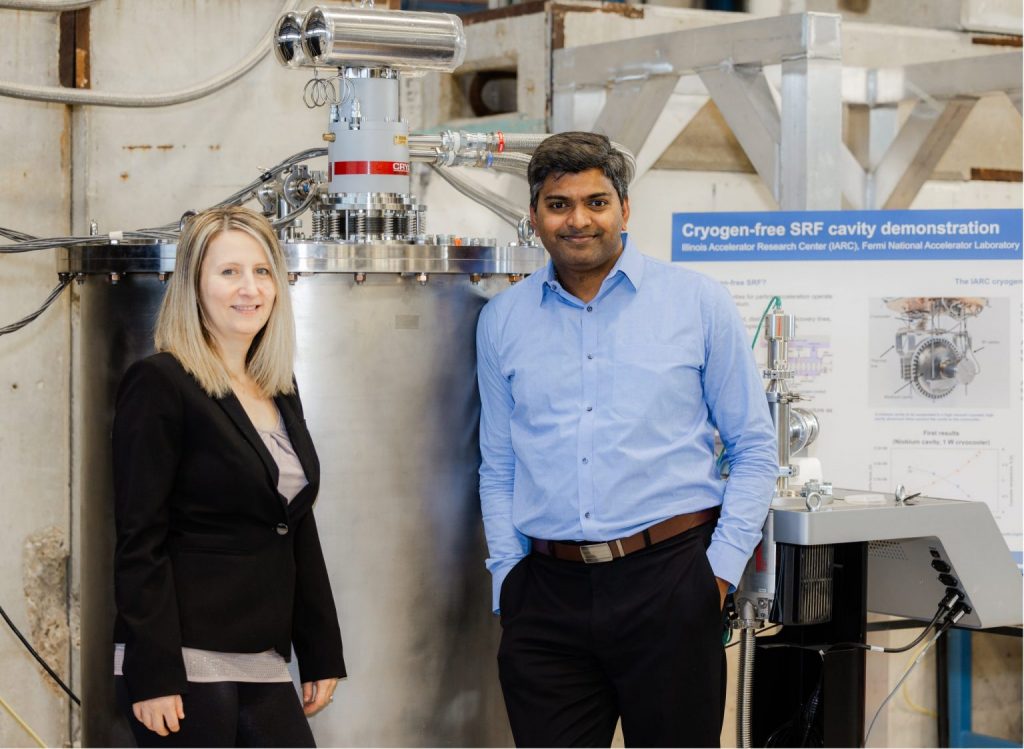
10. Improved the campus and access to it
The year 2024 was a standout for the Fermilab campus as the new Integrated Engineering Research Center, with its environmentally sustainable design, received multiple awards, including the Department of Energy’s 2024 Outstanding Net-Zero Building Program/Project Award and the High Performance Sustainable Building Award. The 80,000-square-foot multi-story laboratory and office building, located next to Wilson Hall, provides workspace for around 100 engineers and technicians and has been bustling with activity since its completion in 2023.
Last year, the Fermilab campus reopened to the public after a hiatus due to the COVID-19 pandemic. In January 2024, Director Lia Merminga announced updates to Fermilab’s site access, including the exciting news that our iconic Wilson Hall had reopened to the public. Since then, thousands of visitors have attended public tours, Saturday Morning Physics lectures, teacher workshops, field trips and other events. Additionally, Lederman Science Center welcomed nearly 6,000 guests. Learn more about visiting the lab here.
In 2024, crews continued improvements on many areas of the Fermilab site, including starting construction on Fermilab’s new welcome center, which is expected to open in fall 2025. Located near Fermilab’s main entrance on Pine Street, the Fermilab Welcome and Access Center will host both informational and administrative functions for smoother processing and access to the site. The construction project also includes a new guardhouse and the reconfiguration of traffic routes for cars, bicyclists and pedestrians to provide easy and secure access to the campus.
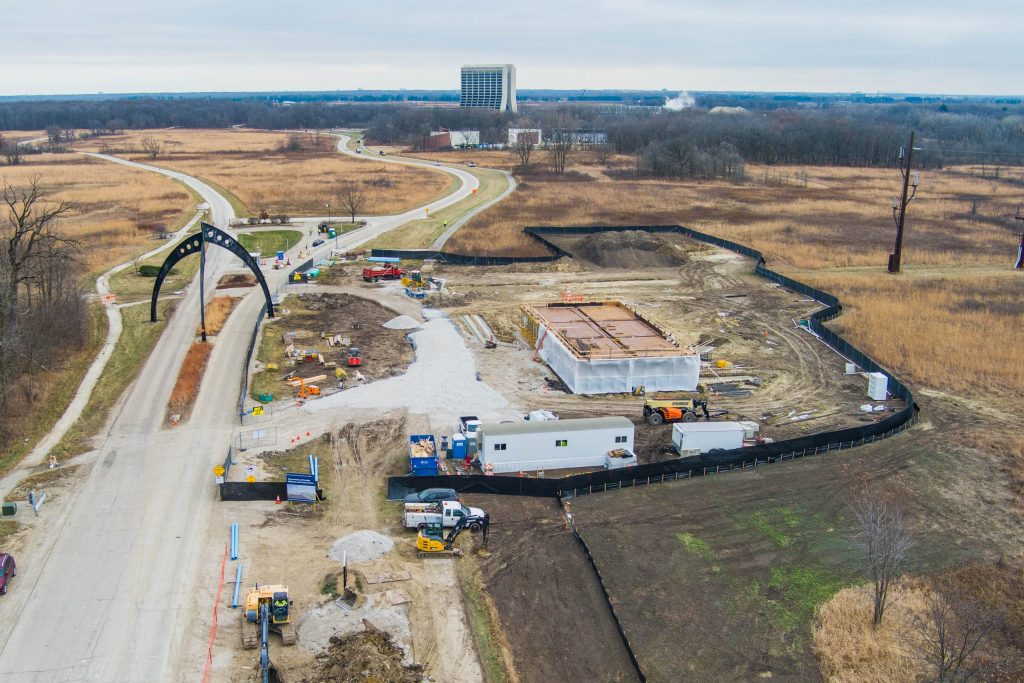
Fermi National Accelerator Laboratory is supported by the Office of Science of the U.S. Department of Energy. The Office of Science is the single largest supporter of basic research in the physical sciences in the United States and is working to address some of the most pressing challenges of our time. For more information, please visit science.energy.gov.
The U.S. Department of Energy’s Fermi National Accelerator Laboratory is expecting a 95-ton coldbox delivery from Sassenage, France. A 55-by-14-foot cryogenic vessel coldbox will soon arrive at the lab after a two-month voyage across the Atlantic Ocean, passage up the Mississippi River and a slow, careful drive via interstate to Batavia, Illinois.
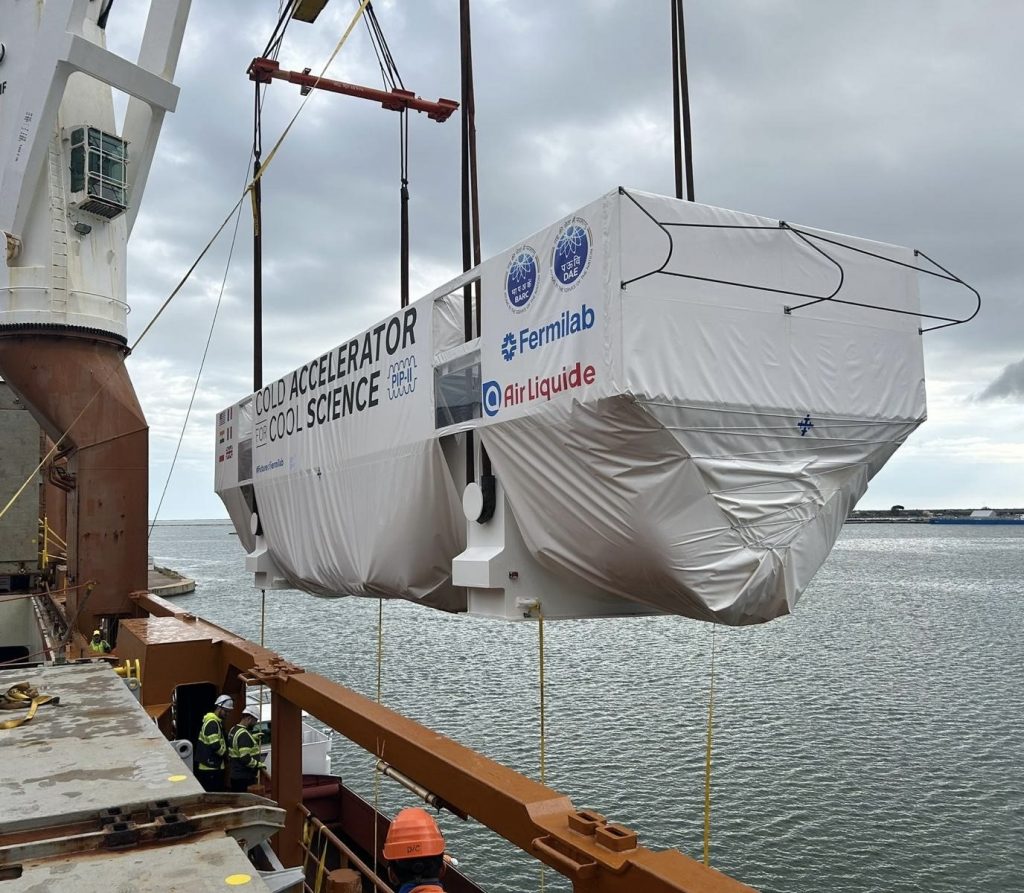
The coldbox will be at the heart of the cryogenic system for the Proton Improvement Plan-II, the project providing significant upgrades to the particle accelerator complex at Fermilab, including a new state-of-the-art linear accelerator. The accelerator will make use of the latest advances in superconducting radio-frequency technologies. But for it to work, the accelerating structures must be kept extremely cold — near the temperature of outer space.
Cryogenics will play a vital role in cooling the superconducting components in the PIP-II accelerator. The coldbox is one piece of the PIP-II cryogenic plant, which is being provided as an in-kind contribution from the Department of Atomic Energy in India — the single largest external contribution to the PIP-II project. The cryogenic plant was built thanks to the combined efforts of the Fermilab and DAE teams on all phases of the project, from technical specifications and design to construction follow-up and, finally, delivery.
The journey
The coldbox, assembled at the Air Liquide Advanced Technologies workshop in Sassenage, France, departed for its journey on Oct. 14. A week later it was loaded onto a transport ship on the southern French coast for its 25-day cross-Atlantic trip to the Port of New Orleans in the United States.
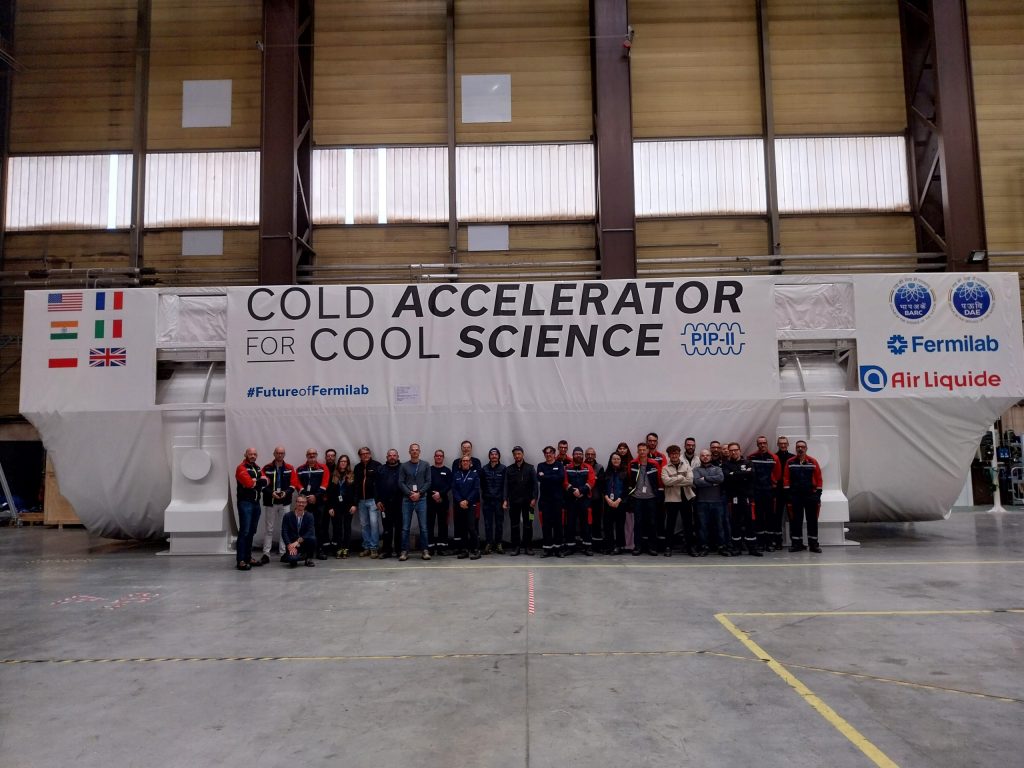
After arrival at the Port of New Orleans in late November, the coldbox was transferred to a river barge for transport up the Mississippi River to Romeoville, Illinois, where it will be offloaded onto a 277-foot-long heavy-haul truck. The coldbox will be driven at 10 miles-per-hour to Fermilab, where it will be carefully moved to a smaller, more maneuverable trailer for the drive across the laboratory campus to the PIP-II site. Crews will install the coldbox inside its new home in the new PIP-II Cryogenic Plant Building.
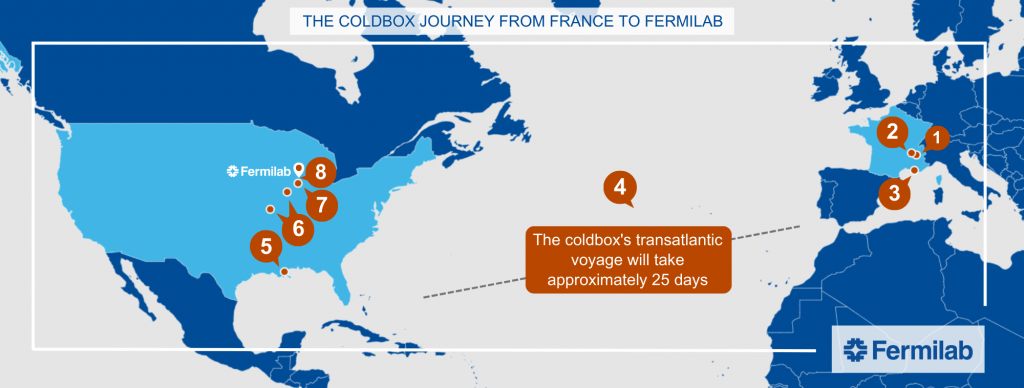
Visitors are invited to Fermilab to see the coldbox delivery from a public viewing area at Wilson Hall. The viewing event is scheduled for January 7 from 9:00 – 11:00 a.m. but is subject to change based on extreme weather. Confirmation or updates on the delivery will be posted to the website 48 hours prior to the event.
The coldbox’s journey to Fermilab was chronicled on the PIP-II website and on Fermilab’s Facebook and X/Twitter. Those interested in attending the January 7 coldbox event should check the website and follow Fermilab on Facebook and X/Twitter for the most up-to-date status of the delivery.
Fermi National Accelerator Laboratory is America’s premier national laboratory for particle physics and accelerator research. Fermi Research Alliance manages Fermilab for the U.S. Department of Energy Office of Science. Visit Fermilab’s website at www.fnal.gov and follow us on social media.
The Proton Improvement Plan-II project, essential for upgrading Fermilab’s particle accelerator complex, just achieved an important milestone by completing its Early Conventional Facilities subproject. This includes infrastructure and building systems to support a new cryogenic plant for PIP-II.
The PIP-II project is constructing a powerful new linear particle accelerator at Fermilab, which will use state-of-the-art superconducting radiofrequency technology to produce the neutrino beam for the Deep Underground Neutrino Experiment. DUNE — the world’s most comprehensive neutrino experiment — is scheduled to start up in 2028. In addition to DUNE, the accelerator upgrade will enable advanced high-energy physics experiments for decades to come.
On Nov. 22, a Department of Energy independent project review team recommended that the PIP-II Early Conventional Facilities subproject is ready for Critical Decision 4 approval. CD-4 is the last of five approval stages — CD-0 through CD-4 — signaling that the Early Conventional Facilities subproject is complete and the start of a series of activities associated with transition to operations. Conventional facilities encompass all the basic and essential systems in a building that allow it to function properly, such as electrical wiring, plumbing and lighting.
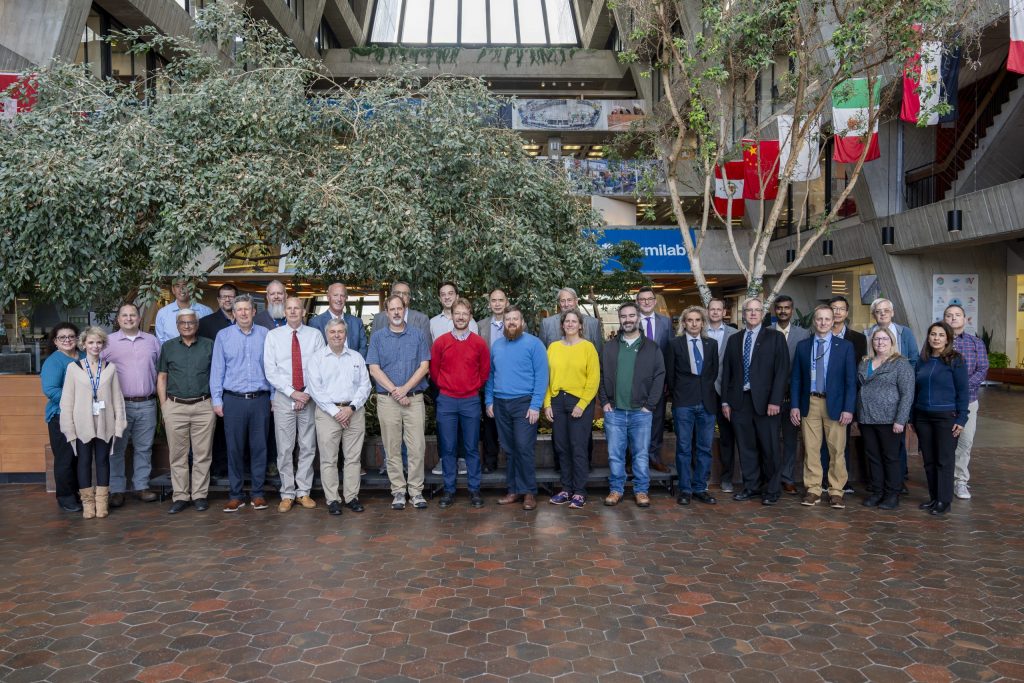
One of the unique aspects of this milestone is proving the success of the subproject model used by the DOE Office of Science. This approach treats each part of an overall project as a standalone unit with its own leadership team, plans and strategy. By granting funding for specific subprojects, DOE facilitates construction to proceed more efficiently.
“The ECF subproject provided an effective means to construct a portion of the PIP-II conventional facilities early, which allowed us to achieve schedule and cost goals,” said Steve Dixon, PIP-II conventional facilities manager. “This was especially challenging because the subproject was constructed during the COVID period. Its success was thanks to close coordination between DOE and Fermilab.”
Cristian Boffo, PIP-II project manager, highlighted the role of the subproject model for enhancing efficiency. “Subprojects are a very effective tool in the project management bag that allows for adaptability as the work is underway,” Boffo explained.
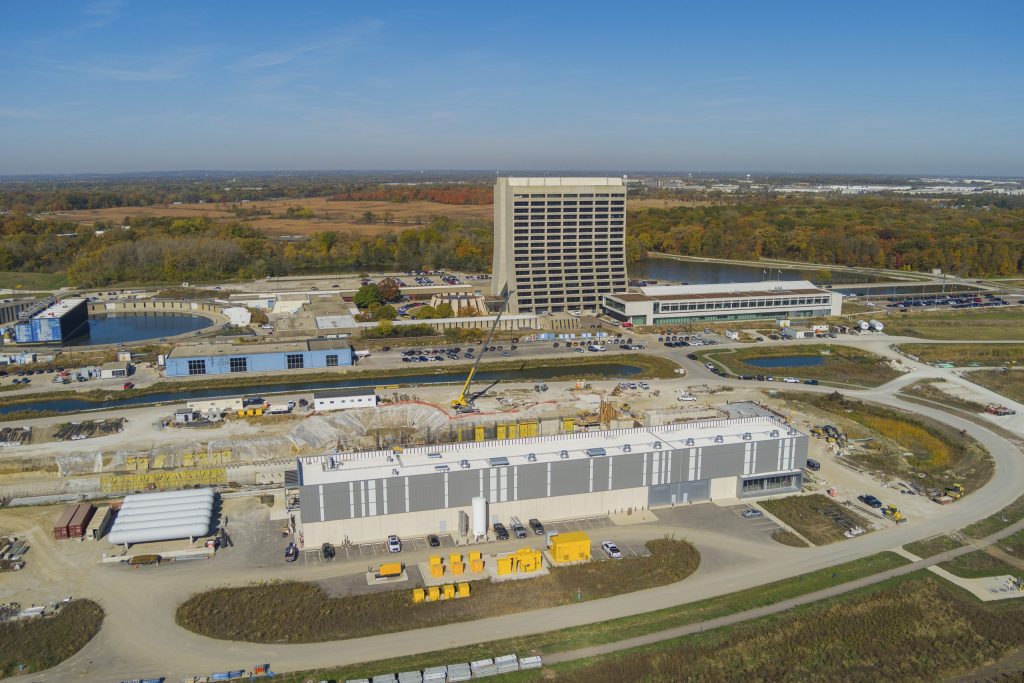
The ECF subproject had four focuses: prepping the site by building roads and rebuilding the cooling pond, constructing the cryogenic plant building, installing the isolated cooling loop, and installing the process power transformer.
The subproject received CD-2/3 approvals from the Department of Energy in July 2020, which authorized Fermilab to start site preparation and construction for the cryogenic plant building. Fermilab received official acceptance of the site work in November 2022, and in April 2023, Fermilab celebrated the completion of the cryogenic plant building.
The cryogenic plant is an in-kind contribution by the Department of Atomic Energy in India — the largest of many in-kind contributions to PIP-II by international partners. An essential piece of the cryogenic plant, the coldbox, was constructed by European industry and is currently en route to Fermilab, scheduled to arrive in late December.
“The PIP-II ECF subproject completion is an important step for Fermilab and the Department of Energy, providing a world-class platform for the global high-energy physics community,” said Steven Neus, federal project director for the Fermi Site Office. “It will enable world-leading discovery experiments investigating the fundamental nature of the universe.”
Fermi National Accelerator Laboratory is supported by the Office of Science of the U.S. Department of Energy. The Office of Science is the single largest supporter of basic research in the physical sciences in the United States and is working to address some of the most pressing challenges of our time. For more information, please visit science.energy.gov.
Since their establishment in 2020, the five U.S. Department of Energy (DOE) National Quantum Information Science Research Centers (NQISRCs) have been expanding the frontier of what’s possible in quantum computing, communication, sensing and materials in ways that will advance basic science for energy, security, communication and logistics. The centers have strengthened the national quantum information science (QIS) ecosystem, achieving scientific and technological breakthroughs as well as training the next-generation quantum workforce.
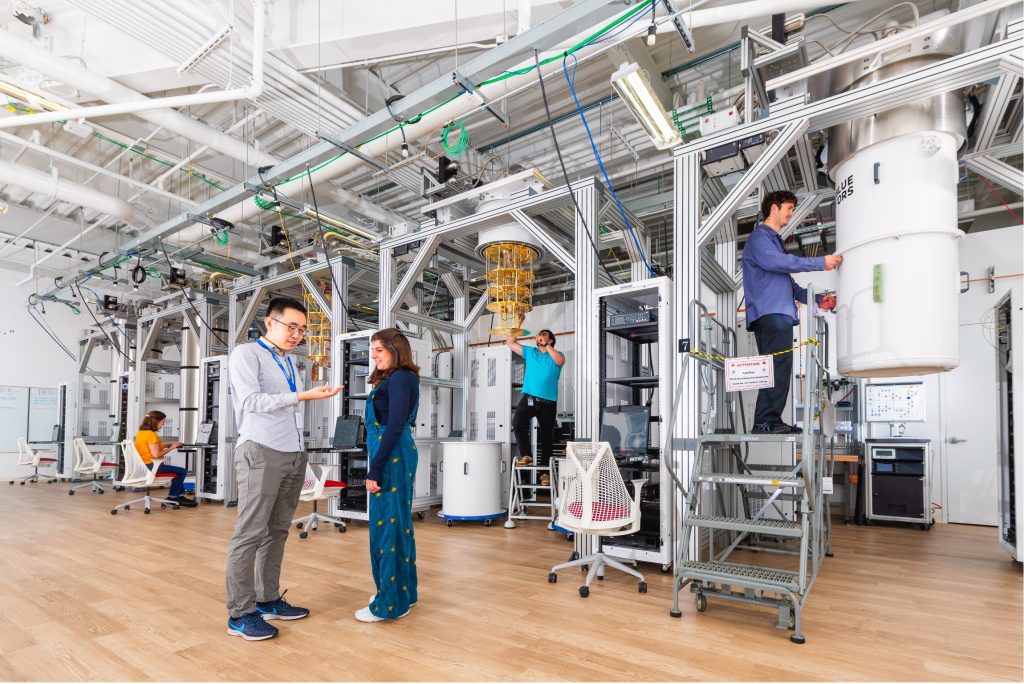
Pictured: SQMS Quantum Garage at Fermilab (Credit: Fermi National Accelerator Laboratory)
Led by a DOE national laboratory, each center comprises a collaborative team spanning a broad range of scientific and engineering disciplines. Bringing together national laboratories, universities and tech companies, the centers have developed into a strong community by creating bridges between the world’s top experts in multiple scientific and technological fields. This further enables researchers to solve major cross-cutting challenges in QIS.
Together, they unite more than 1,500 experts across 115 institutions in North America and Europe. The five centers are:
- Co-design Center for Quantum Advantage (C2QA) led by Brookhaven National Laboratory
- Q-NEXT, led by Argonne National Laboratory
- Quantum Science Center (QSC) led by Oak Ridge National Laboratory
- Quantum Systems Accelerator (QSA) led by Lawrence Berkeley National Laboratory
- Superconducting Quantum Materials and Systems Center (SQMS) led by Fermi National Accelerator Laboratory
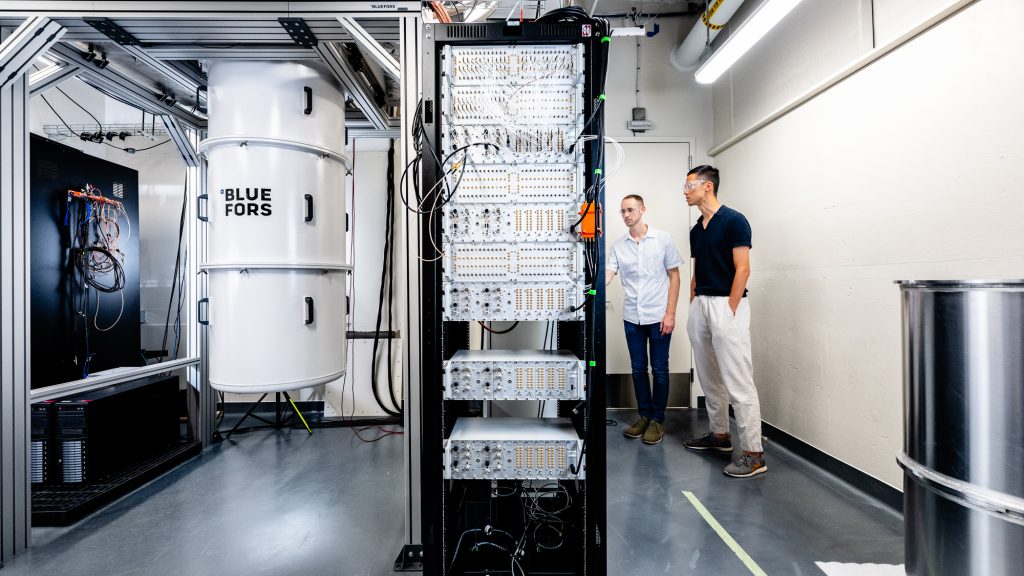
Pictured: Collaborators work at the quantum computing test bed at the Argonne Quantum Foundry. (Credit: Argonne National Laboratory)
In their four years of growth and operation, the NQISRCs have achieved multiple successes in QIS and technology:
- Advanced the fundamental science and understanding of the physics behind quantum devices.
- Enhanced quantum devices, the building blocks of quantum computers and sensors, to record performance levels through innovative materials science.
- Built and deployed new quantum processors and sensors at DOE national labs, universities and industry partners.
- Tailored and designed algorithms to efficiently process data on novel quantum devices while exploring commercial quantum platforms and evaluating the computational capabilities of different hardware at DOE’s quantum testbeds.
- Built unique, cutting-edge facilities and instrumentation to characterize, measure, integrate and use quantum devices, processors and sensors.
- Trained more than 1,000 students and early-career researchers through summer schools, internships and research experiences at facilities affiliated with the centers.
- Launched the U.S. Quantum Information Science Summer School, the first of its kind to facilitate an interconnected quantum ecosystem across academia, national labs and industry to train and grow a quantum-ready workforce.
- Connected over 1,600 job seekers with hiring managers from national labs, academia and industry at the virtual Quantum Information Science Career Fair.
- Unveiled nqisrc.org, a centralized digital home for the five centers. The site outlines the NQISRCs’ scientific accomplishments, research developments, workforce opportunities, and educational and scientific resources.
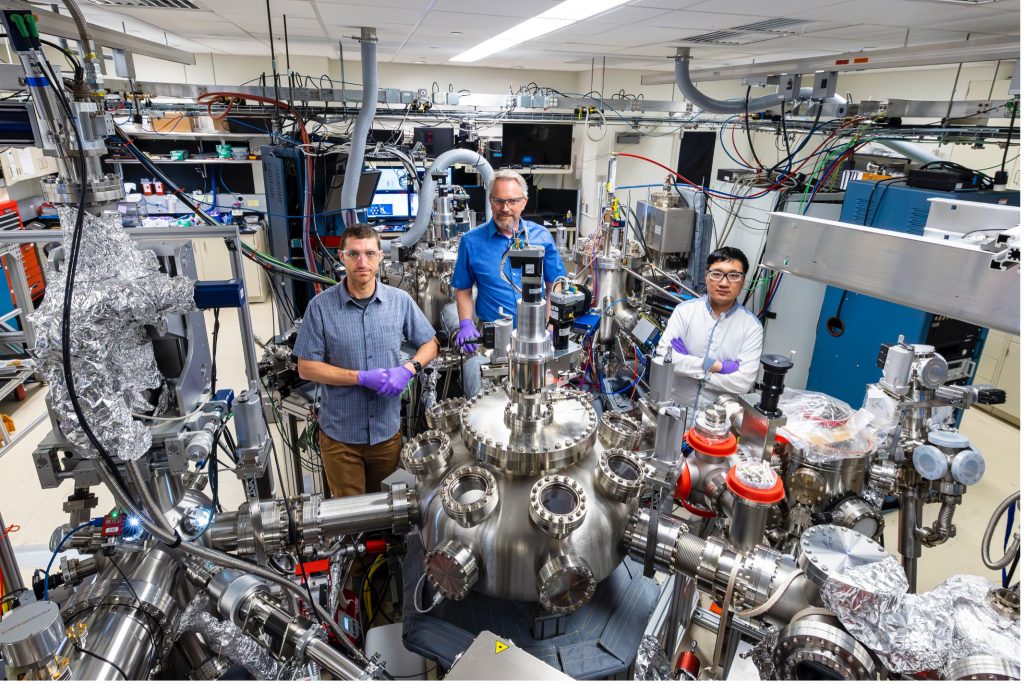
Pictured: The Quantum Thin Film and Characterization Lab at Oak Ridge National Laboratory where QSC researchers combine molecular beam epitaxy for quantum thin film and heterostructure synthesis with characterization tools to better understand materials at the level of atoms and electrons. This lab is central to the QSC’s efforts to develop quantum material platforms for quantum computing and sensing applications. In this image, Matthew Brahlek, Robert Moore and Qiangsheng Lu are developing topological superconducting materials for quantum computing applications in support of the Quantum Science Center headquartered at Oak Ridge National Laboratory. (Credit: Carlos Jones, Oak Ridge National Laboratory)
In fall 2024, 200 experts from the five centers met to review four years of progress and discuss an expanded vision for the future of QIS. The event fostered collaboration and strengthened cross-center partnerships.
By bringing quantum technologies to a broader set of researchers and users, the centers are fulfilling DOE’s mission to advance science for the benefit of society.
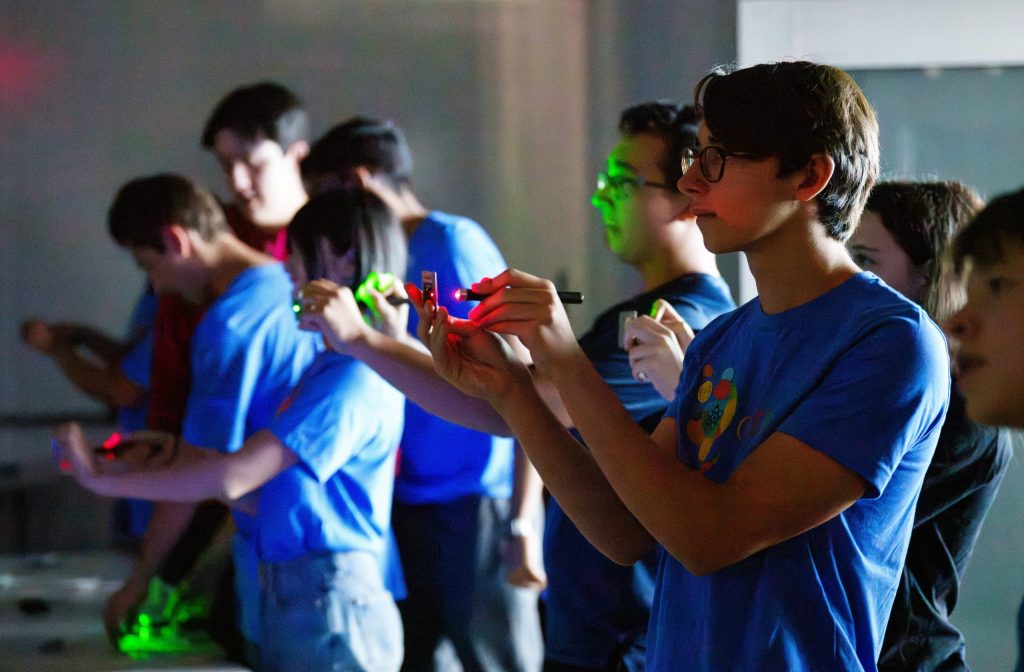
Pictured: As part of this coordinated effort, the Quantum Systems Accelerator and its partners have expanded summer school programs for high school teachers and students, collaborating to share advancements in QIS. Students replicated the classic Young’s double-slit experiment at the 2024 QCaMP in Albuquerque, New Mexico, demonstrating how laser light waves interfere as they pass through two apertures. (Credit: Sandia National Laboratories)
The five NQISRCs have grown into a unique and well-connected network of experts and facilities, which together will be critical to advancing the burgeoning field of QIS in the years ahead.
Commenting on the successes of the NQISRCs, the Department of Energy’s Deputy Director of Science Programs Harriet Kung said, “As we mark four years of extraordinary progress, the five National Quantum Information Science Research Centers are not only continuing to push the boundaries of quantum information science but are building a robust ecosystem that spans academia, national labs and industry. Their groundbreaking work in quantum computing, sensing, communication and materials is shaping the future of technology, security and workforce development in ways that will benefit society and meet DOE’s mission to advance science for the public good.”
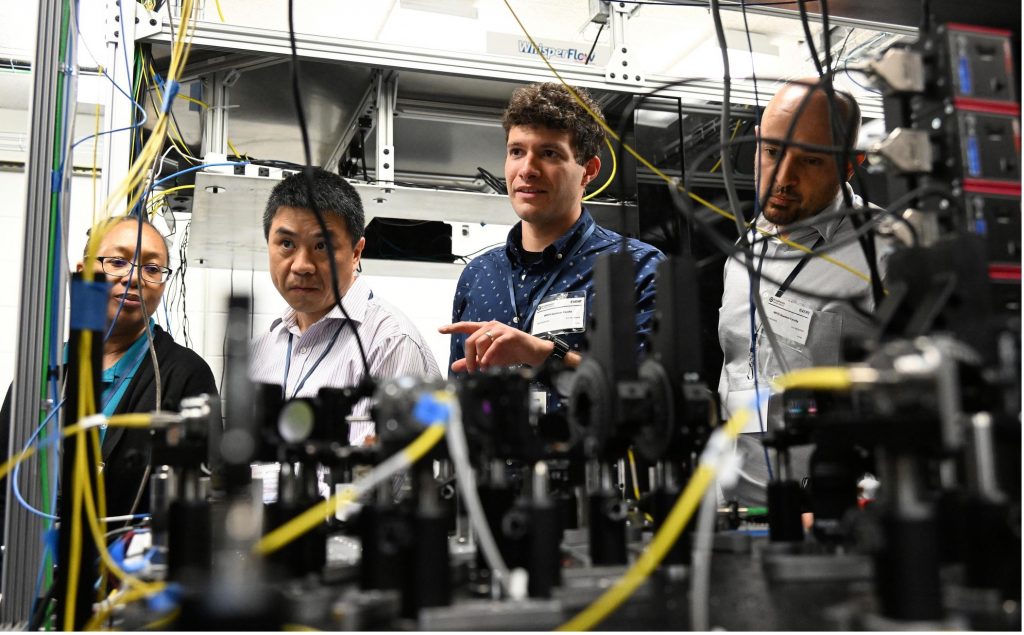
Pictured: During the Faculty Outreach for Quantum-Invested UniversitieS (FOQUS), program, participants toured the Quantum Network Facility at Brookhaven Lab. (Credit: Kevin Coughlin, Brookhaven National Laboratory)
The Superconducting Quantum Materials and Systems Center at Fermilab is supported by the DOE Office of Science.
The Superconducting Quantum Materials and Systems Center is one of the five U.S. Department of Energy National Quantum Information Science Research Centers. Led by Fermi National Accelerator Laboratory, SQMS is a collaboration of more than 30 partner institutions — national labs, academia and industry — working together to bring transformational advances in the field of quantum information science. The center leverages Fermilab’s expertise in building complex particle accelerators to engineer multiqubit quantum processor platforms based on state-of-the-art qubits and superconducting technologies. Working hand in hand with embedded industry partners, SQMS will build a quantum computer and new quantum sensors at Fermilab, which will open unprecedented computational opportunities. For more information, please visit sqmscenter.fnal.gov.
The Quantum Systems Accelerator (QSA) is one of the five National Quantum Information Science Research Centers funded by the U.S. Department of Energy Office of Science. Led by Lawrence Berkeley National Laboratory (Berkeley Lab) and with Sandia National Laboratories as lead partner, QSA catalyzes national leadership in quantum information science to co-design the algorithms, quantum devices, and engineering solutions needed to deliver certified quantum advantage in scientific applications. QSA brings together dozens of scientists who are pioneers of many of today’s unique quantum engineering and fabrication capabilities. In addition to industry and academic partners across the world, 15 institutions are part of QSA: Lawrence Berkeley National Laboratory, Sandia National Laboratories, University of Colorado at Boulder, MIT Lincoln Laboratory, Caltech, Duke University, Harvard University, Massachusetts Institute of Technology, Tufts University, UC Berkeley, University of Maryland, University of New Mexico, University of Southern California, UT Austin, and Canada’s Université de Sherbrooke. For more information, please visit quantumsystemsaccelerator.org.
Q-NEXT is a U.S. Department of Energy National Quantum Information Science Research Center led by Argonne National Laboratory. Q-NEXT brings together world-class researchers from national laboratories, universities and U.S. technology companies with the goal of developing the science and technology to control and distribute quantum information. Q-NEXT collaborators and institutions have established two national foundries for quantum materials and devices, develop networks of sensors and secure communications systems, establish simulation and network test beds, and train the next-generation quantum-ready workforce to ensure continued U.S. scientific and economic leadership in this rapidly advancing field. For more information, visit q-next.org.
The Co-design Center for Quantum Advantage (C2QA) is one of the five National Quantum Information Science Research Centers funded by the U.S. Department of Energy Office of Science. Led by DOE’s Brookhaven National Laboratory, C2QA aims to overcome the limitations of today’s noisy intermediate-scale quantum computer systems by co-designing quantum materials, devices, and software and algorithms. The C2QA team includes more than 350 researchers from national labs, academia, and industry working together to achieve quantum advantage for scientific computations in high energy, nuclear, chemical and condensed matter physics. For more information, visit www.bnl.gov/quantumcenter.
The QSC, a DOE National Quantum Information Science Research Center led by ORNL, performs cutting-edge research at national laboratories, universities, and industry partners to overcome key roadblocks in quantum state resilience, controllability, and ultimately the scalability of quantum technologies. QSC researchers are designing materials that enable topological quantum computing; implementing new quantum sensors to characterize topological states and detect dark matter; and designing quantum algorithms and simulations to provide a greater understanding of quantum materials, chemistry, and quantum field theories. These innovations enable the QSC to accelerate information processing, explore the previously unmeasurable, and better predict quantum performance across technologies. For more information, visit qscience.org.