Silicon is a material widely used in computing: It is used in computer chips, circuits, displays and other modern computing devices. Silicon is also used as the substrate, or the foundation of quantum computing chips.
Researchers at the Superconducting Quantum Materials and Systems Center, hosted by the U.S. Department of Energy’s Fermi National Accelerator Laboratory, demonstrated that silicon substrates could be detrimental to the performance of quantum processors. SQMS Center scientists have measured silicon’s effect on the lifespan of qubits with parts-per-billion precision. These findings have been published in Physical Review Applied.
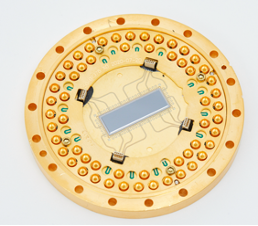
A superconducting-based quantum processor, composed of several thin film materials deposited on top of a silicon substrate. Photo: Rigetti Computing
New approaches to computing
Calculations once performed on pen and paper have since been handed to computers. Classical computers rely on bits, 1 or 0, which have limitations. Quantum computers offer a new approach to computing that relies on quantum mechanics. These novel devices could perform calculations that would take years or be practically impossible for a classical computer to perform.
Using the power of quantum mechanics, qubits—the basic unit of quantum information held within a quantum computing chip—can be both a 1 and a 0 at the same time. Processing and storing information in qubits is challenging and requires a well-controlled environment. Small environmental disturbances or flaws in the qubit’s materials can destroy the information.
Qubits require near-perfect conditions to maintain the integrity of their quantum state, and certain material properties can decrease the qubit lifespan. This phenomenon, called quantum decoherence, is a critical obstacle to overcome to operate quantum processors.
Disentangling the architecture
The first step to reduce or eliminate quantum decoherence is to understand its root causes. SQMS Center scientists are studying a broadly used type of qubit called the transmon qubit. It is made of several layers of different materials with unique properties. Each layer, and each interface between these layers, play an important role in contributing to quantum decoherence. They create “traps” where microwave photons—key in storing and processing quantum information—can be absorbed and disappear.
Researchers cannot unequivocally distinguish where the traps are located or which of the various materials or interfaces are driving decoherence based on the measurement of the qubit alone. Scientists at the SQMS Center use uniquely sensitive tools to study these effects from the materials that make up the transmon qubits.
“We are disentangling the system to see how individual sub-components contribute to the decoherence of the qubits,” said Alexander Romanenko, Fermilab’s chief technology officer, head of the Applied Physics and Superconducting Technology Division and SQMS Center quantum technology thrust leader. “A few years ago, we realized that our [superconducting radio frequency] cavities could be tools to assess microwave losses of these materials with a preciseness of parts-per-billion and above.”
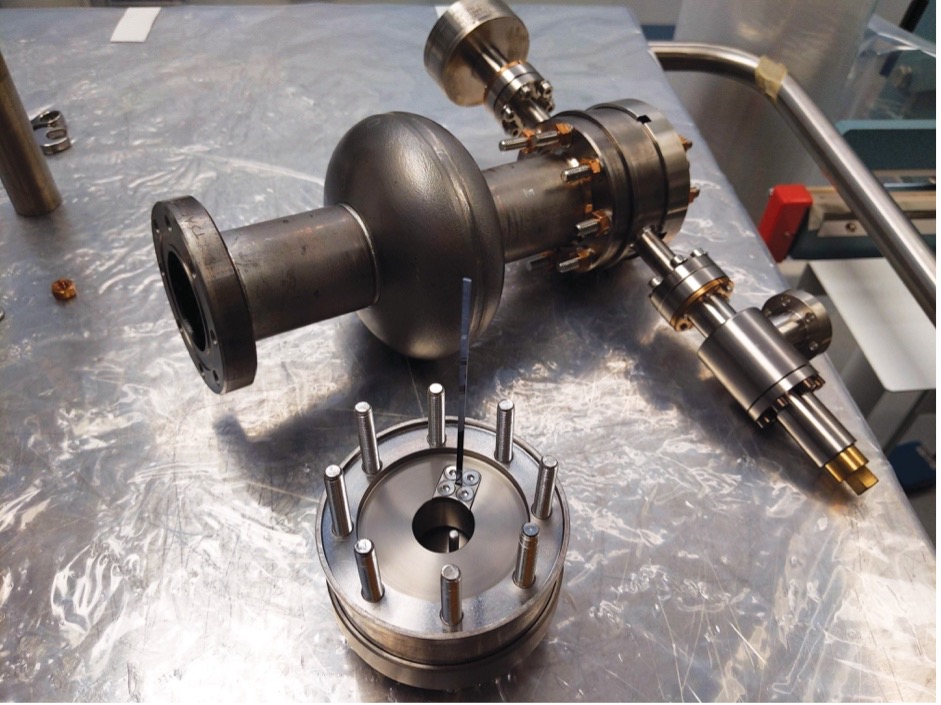
The silicon sample connected to the holder appears in the foreground, while the SRF cavity used in the study rests in the background. Photo: SQMS Center
Measurements at cold temperatures
SQMS Center researchers have directly measured the loss tangent—a material’s ability to absorb electromagnetic energy—of high-resistivity silicon. These measurements were performed at temperatures only hundreds of a degree above absolute zero. These cold temperatures offer the right conditions for superconducting transmon qubits to operate.
“The main motivation for why we did this experiment was that there were no direct measurements on this loss tangent at such low temperatures,” said Mattia Checchin, SQMS Center scientist and the lead researcher on this project.
No material is perfect. Through rigorous testing and studies, researchers are building a more comprehensive understanding of the materials and properties best suited for quantum computing.
Checchin cooled a metallic niobium SRF cavity in a dilution refrigerator and filled it with a standing electromagnetic wave. After placing a sample of silicon inside the cavity, Checchin compared the time the wave dissipated without the silicon present to the time with it present. He found that the waves dissipated more than 100 times faster with the silicon present—from 100 milliseconds without silicon to less than a millisecond with it.
“The silicon dissipation we measured was an order of magnitude worse than the number widely reported in the [quantum information science] field,” said Anna Grassellino, director of the SQMS Center. “Our approach of disentangling the problem by studying each qubit sub-component with uniquely sensitive tools has shown that the contribution of the silicon substrate to decoherence of the transmon qubit is substantial.”
Re-evaluating silicon
Companies developing quantum computers based on quantum computing chips often use silicon as a substrate. SQMS Center studies highlight the importance of understanding which of silicon’s properties have negative effects. This research also helps define specifications for silicon that would ensure that substrates are useful. Another option is to substitute the silicon with sapphire or another less lossy material.
“Sapphire, in principle, is like a perfect insulator—so much better than silicon,” said Checchin. “Even sapphire has some losses at really low temperatures. In general, you would like to have a substrate that is lossless.”
Researchers often use the same techniques for fabricating silicon-based microelectronic devices to place qubits on silicon substrate. So sapphire has rarely been used for quantum computing.
“It has taken years of material science and device physics studies to develop the niobium material specifications that would ensure consistently high-performances in SRF cavities,” said Romanenko. “Similar studies need to be done for materials that comprise superconducting qubits. This effort includes researchers working together with the material industry vendors.”
Regardless of which material is used for qubits, eliminating losses and increasing coherence time is crucial to the success of quantum computing. No material is perfect. Through rigorous testing and studies, researchers are building a more comprehensive understanding of the materials and properties best suited for quantum computing.
This loss tangent measurement is a substantial step forward in the search for the best materials for quantum computing. SQMS Center scientists have isolated a problem and can now explore whether a more refined version of silicon or sapphire will harness the computational power of a qubit.
The Superconducting Quantum Materials and Systems Center is one of the five U.S. Department of Energy National Quantum Information Science Research Centers. Led by Fermi National Accelerator Laboratory, SQMS is a collaboration of 23 partner institutions—national labs, academia and industry—working together to bring transformational advances in the field of quantum information science. The center leverages Fermilab’s expertise in building complex particle accelerators to engineer multiqubit quantum processor platforms based on state-of-the-art qubits and superconducting technologies. Working hand in hand with embedded industry partners, SQMS will build a quantum computer and new quantum sensors at Fermilab, which will open unprecedented computational opportunities. For more information, please visit sqms.fnal.gov.
Fermi National Accelerator Laboratory is supported by the Office of Science of the U.S. Department of Energy. The Office of Science is the single largest supporter of basic research in the physical sciences in the United States and is working to address some of the most pressing challenges of our time. For more information, please visit science.energy.gov.