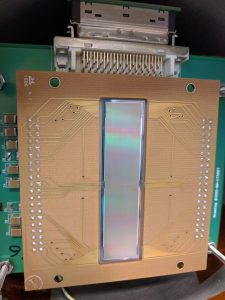
SENSEI’s innovative sensors, called skipper CCDs, make it possible to look for low-mass dark matter. Photo courtesy of Javier Tiffenberg
Technology proposed 30 years ago to search for dark matter is finally seeing the light.
Scientists are using innovative sensors, called skipper CCDs (short for charge-coupled devices) in a new type of dark matter detection project. Scientists will use the project, known as SENSEI, to find the lightest dark matter particles anyone has ever looked for.
Dark matter — so named because it doesn’t absorb, reflect or emit light — constitutes 27 percent of the universe, but the jury is still out on what it’s made of. The primary theoretical suspect for the main component of dark matter is a particle scientists have descriptively named the weakly interactive massive particle, or WIMP.
But since none of these heavy particles, which are expected to have a mass 100 times that of a proton, have shown up in experiments, it might be time for researchers to think small.
“There is a growing interest in looking for different kinds of dark matter that are additives to the standard WIMP model,” said Fermilab scientist Javier Tiffenberg, a leader of the SENSEI collaboration. “Lightweight, or low-mass, dark matter is a very compelling possibility, and for the first time, the technology is there to explore these candidates.”
Low-mass dark matter would leave a tiny, difficult-to-see signature when it collides with material inside a detector. Catching these elusive particles requires a dark-matter-detecting master: SENSEI.
Sensing the unseen
In traditional dark matter experiments, scientists look for a transfer of energy that would occur if dark matter particles collided with an ordinary nucleus, but SENSEI is different. It looks for direct interactions of dark matter particles colliding with electrons.
“That is a big difference — you get a lot more energy transferred in this case because an electron is so light compared to a nucleus,” Tiffenberg said.
If dark matter has low mass — much smaller than the WIMP model suggests — then it would be many times lighter than an atomic nucleus. So if it were to collide with a nucleus, the resulting energy transfer would be far too small tell us anything. It would be like throwing a ping pong ball at a boulder: the heavy object isn’t going anywhere, and there would be no sign the two had come into contact.
An electron is nowhere near as heavy as an atomic nucleus. In fact, a single proton has about 1,836 times more mass than an electron. So the collision of a low-mass dark matter particle with an electron has a much better chance of leaving a mark — more bowling ball than the nucleus’s boulder.
Even so, the electron is still a bowling ball compared to the low-mass dark matter particle. An energy transfer between the two would leave only a blip of energy, one either too small for most detectors to pick up or easily overshadowed by noise in the data. There is a small exchange of energy, but, if the detector isn’t sensitive enough, it could appear as though nothing happens.
“The bowling ball will move a very tiny amount,” said Fermilab scientist Juan Estrada, a SENSEI collaborator. “You need a very precise detector to see this interaction of lightweight particles with something that is much heavier.”
That’s where SENSEI’s sensitive skipper CCDs come in: They will pick up on that tiny transfer of energy.
CCDs have been used for other dark matter detection experiments, such as the Dark Matter in CCDs (or DAMIC) experiment operating at SNOLAB in Canada. These CCDs were a spinoff from sensors developed for use in the Dark Energy Camera in Chile and other dark energy search projects.
CCDs are typically made of silicon divided into pixels. When a dark matter particle passes through the CCD, it collides with silicon’s electrons, knocking them free, leaving a net electric charge in each pixel the particle passes through. The electrons then flow through adjacent pixels and are ultimately read as a current in a device that measures the number of electrons freed from each CCD pixel. That measurement tells scientists about the mass and energy of the particle — in this case the dark matter particle — that got the chain reaction going. A massive particle, like a WIMP, would free a gusher of electrons, but a low-mass particle might free only one or two.
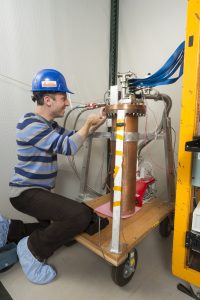
Fermilab scientist Javier Tiffenberg makes adjustments to the SENEI prototype, which is located in a cavern 385 feet below ground. Photo: Reidar Hahn
Typical CCDs can measure the charge left behind only once, which makes it difficult to decide if a tiny energy signal from one or two electrons is real or an error.
Skipper CCDs are a new generation of the technology that helps eliminate the “iffiness” of a measurement that has a one- or two-electron margin of error. That allows for much higher precision thanks to a unique design.
“In the past, detectors could measure the amount of charge of the energy deposited in each pixel only once,” Tiffenberg said. “The big step forward for the skipper CCD is that we are able to measure this charge as many times as we want.”
The charge left behind in the skipper CCD by dark matter knocking electrons free can be sampled multiple times and then averaged, a method that yields a more precise measurement of the charge deposited in each pixel than the measure-one-and-done technique. That’s the rule of statistics: With more data, you get closer to a property’s true value.
SENSEI scientists take advantage of the skipper CCD architecture, measuring the number of electrons in a single pixel a whopping 4,000 times and then averaging them. That minimizes the measurement’s error — or noise — and clarifies the signal.
“This is a simple idea, but it took us 30 years to get it to work,” Estrada said.
From idea, to reality, to beyond
A small SENSEI prototype is currently running at Fermilab in a detector hall 385 feet below ground, and it has demonstrated that this detector design will work in the hunt for dark matter.
After a few decades existing as only an idea, skipper CCD technology and SENSEI were brought to life by Laboratory Directed Research and Development (LDRD) funds at Fermilab and Lawrence Berkeley National Laboratory (Berkeley Lab). The Fermilab LDRDs were awarded only recently — less than two years ago — but close collaboration between the two laboratories has already yielded SENSEI’s promising design, partially thanks to Berkeley lab’s previous work in skipper CCD design.
Fermilab LDRD funds allow researchers to test the sensors and develop detectors based on the science, and the Berkeley Lab LDRD funds support the sensor design, which was originally proposed by Berkeley Lab scientist Steve Holland.
“It is the combination of the two LDRDs that really make SENSEI possible,” Estrada said.
LDRD programs are intended to provide funding for development of novel, cutting-edge ideas for scientific discovery, and SENSEI technology certainly fits the bill — even beyond its search for dark matter.
Future SENSEI research will also receive a boost thanks to a recent grant from the Heising-Simons Foundation.
“SENSEI is very cool, but what’s really impressive is that the skipper CCD will allow the SENSEI science and a lot of other applications,” Estrada said. “Astronomical studies are limited by the sensitivity of their experimental measurements, and having sensors without noise is the equivalent of making your telescope bigger — more sensitive.”
SENSEI technology may also be critical in the hunt for a fourth type of neutrino, called the sterile neutrino, which seems to be even more shy than its three notoriously elusive neutrino family members.
A larger SENSEI detector equipped with more skipper CCDs will be deployed within the year. It’s possible it might not detect anything, sending researchers back to the drawing board in the hunt for dark matter. Or SENSEI might finally make contact with dark matter — and that would be SENSEI-tional.