Earlier this month, the Muon g-2 (“g minus two”) experiment at Fermilab began its second run to search for hidden particles and forces.
Over the next three months, scientists expect to accumulate double the amount of data collected in Run 1 and make the world’s most precise measurement of the muon’s anomalous magnetic moment, often expressed as the quantity g-2.
Run 2 features several improvements that scientists made to the experiment over the past eight months.
“We’re looking to have a more stable environment in which we take the data, because in the first data-taking period we were trying to get things working and evaluating how they’re working,” said Mark Lancaster, the experiment’s co-spokesperson and a professor of physics at the University of Manchester and University College London. “Now we’re trying to move into the mode where things are much more stable, and we can run for a reasonable period of time without any interventions.”
Muons are elementary particles similar to, but much heavier than, electrons. A muon’s magnetic moment — a characteristic related to the orientation and strength of its internal magnet — changes as it spins, an effect called precession. Lancaster and his colleagues are measuring the precession frequency of the magnetic moment very precisely and comparing the result to what theorists predict it should be. In doing so, they hope to confirm, or even revise, the Standard Model of particle physics.
“As it travels through the universe, a particle is never really strictly alone,” said Fermilab’s Chris Polly, the experiment’s other co-spokesperson. “There’s constantly an entourage of other particles that appear out of the vacuum. They come out of nowhere, and they disappear just as quickly as they popped into existence.”
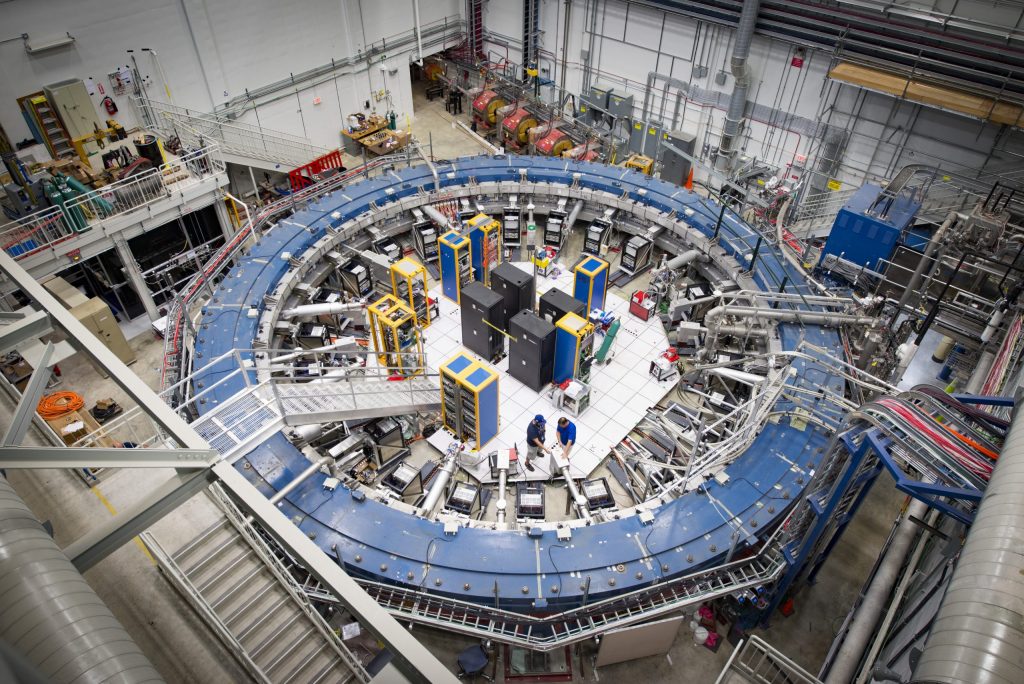
The Muon g-2 experiment recently started its second run. Scientists use this particle storage, a 50-foot-diameter magnet, to look for hidden particles and forces. Photo: Reidar Hahn
Those particles slightly change the muon’s magnetic moment. By calculating how often they will pop in and out of the vacuum and interact with the muon, scientists can predict the impact of all the known particles on the magnetic moment to very high precision. The comparison of this prediction with the experimentally obtained value will tell scientists whether there are additional, undiscovered particles or forces that change the magnetic moment.
At rest, muons decay in just two millionths of a second. That decay produces two neutrinos and a positron, which is a positively charged electron.
“The bulk of our data come from looking at energies and times of decay positrons that came from the muons,” said Brendan Kiburg, a Fermilab particle physicist involved in the experiment.
Getting that data requires a very uniform, precisely measured magnetic field.
“It’s incredibly important that we know the magnetic field the muons are experiencing,” Kiburg said. “Since the new physics that we’re looking for is embedded in the precession frequency, you have to make sure that the muons don’t see a different magnetic field than the one we’re measuring.”
Fine-tuning the ring
The experiment’s storage ring magnet came to Fermilab from its original home at Brookhaven National Laboratory in 2013. After years of construction and adjustments, operators got the beam tuned up and engaged in Run 1, a three-month production run in 2018.
“Because of that production run, we were able to learn about a few deficiencies that we really needed to fix,” Polly said.
There are several areas the team focused on over the summer. The first was a system of quadrupole magnets that focus the muons and prevent them from spiraling up or down.
“We discovered during the shutdown that we needed to improve the reliability of the quadrupoles’ operation, especially at the higher voltages that we would like to achieve in the upcoming run,” Polly said.
Another issue involved a device called an electromagnetic kicker. It shifts the muons’ orbit very slightly to keep them on a path that stays inside the ring.
“The kicker is probably the single most important component of the experiment beyond the ring itself,” Kiburg said.
Without the kicker, the muons behave like a Formula One driver whose racecar is at the wrong angle, sending them careening into the wall on the first lap. To avoid this, the kicker shifts the angle of the muons as they come through the ring’s gate.
“One of the issues with the kicker at Brookhaven was that it was too slow,” Polly said. “Instead of giving the muons a kick on the first turn and shutting off, the kicker pulse continued for two or three revolutions around the ring. That was less than ideal, so we designed a kicker for this experiment that could be up and back down in a single turn.”
While the kick deployed during Run 1 at Fermilab was three times faster, it wasn’t strong enough to push the muons into precisely the perfect orbit around the ring. During the shutdown, the team upgraded the ring to accommodate a more powerful kicker.
The third problem was the temperature control in the Muon g-2 building. The magnetic storage-ring is extremely sensitive to temperature — so much so that a change of more than a single degree Celsius can cause it to expand or contract, degrading the magnetic field. While performing Run 1 during the hottest summer months, maintaining the facility’s temperature was a challenge. Improvements to the facility’s heating and cooling systems should fix that, Polly said.
A mountain of data
The team recently began bringing beam to the storage ring and testing that the upgrades worked as planned. A key goal of Run 2 is to measure the magnetic moment very precisely, to 70 parts per billion. To get that kind of precision, the magnetic field must be highly uniform.
“We were able to adjust the magnetic field so that it’s two to three times more uniform,” Polly said. “So, though we’re using the same container, we’ve in fact turned it into a much better container in terms of understanding this magnetic field.”
The team also had to boost the experiment’s muon flux, the number of muons per second required to reach the necessary statistical precision. In Run 1, they achieved about half of their goal. A bevy of upgrades completed over the summer is expected to increase the flux to about 75 percent of the goal. A final upgrade the team is considering for next summer would get the flux the rest of the way, Polly said.
One upcoming challenge is the sheer volume of data. Run 2 aims to reduce the uncertainty in the result from the Brookhaven Muon g-2 experiment by a factor of four, which requires 16 times the statistics. That’s a lot of data.
“Our aim is to process the data as it arrives,” Lancaster said. “We’re using distributed computing for everything, so we process everything on the grid. Part of what we’re striving to do is to make that more robust and reliable.”
And robustness and reliability require rigor.
“This is why you go through the whole design process so carefully,” Kiburg said. “It’s so you can get to a point where you are turning it into a physics result, and we are on the doorstep there, so this is a fun time.”
The Muon g-2 experiment is supported by DOE’s Office of Science.