Batavia, Ill. — Using detectors chilled to near absolute zero, from a vantage point half a mile below ground, physicists of the Cryogenic Dark Matter Search today (November 12) announced the launch of a quest that could lead to solving two mysteries that may turn out to be one and the same: the identity of the dark matter that pervades the universe, and the existence of supersymmetric particles predicted by particle physics theory. Scientists of CDMS II, an experiment managed by the Department of Energy’s Fermi National Accelerator Laboratory hope to discover WIMPs, or weakly interacting massive particles, the leading candidates for the constituents of dark matter-which may be identical to neutralinos, undiscovered particles predicted by the theory of supersymmetry.
“There’s this arrow from particle physics and this arrow from cosmology and they seem to be pointing to the same place,” said Case Western Reserve University’s Dan Akerib, deputy project manager of CDMS II. “Detection of a neutralino would be very big for cosmology and it would also be very big for particle physics.”
The CDMS II experiment, a collaboration of scientists from 12 institutions with support from DOE’s Office of Science and the National Science Foundation, uses a detector located deep underground in the historic Soudan Iron Mine in northeastern Minnesota. Experimenters seek signals of WIMPs, particles much more massive than a proton but interacting so weakly with other particles that thousands would pass through a human body each second without leaving a trace.
Remarkably, in the kind of convergence that gets physicists’ attention, the characteristics of this cosmic missing matter particle now appear to match those of the supersymmetric neutralino.
“Either that is a cosmic coincidence, or the universe is telling us something,” said Fermilab’s Dan Bauer, CDMS project manager.
By watching how galaxies spin-how gravity affects their contingent stars-astronomers have known for 70 years that the matter we see cannot constitute all the matter in the universe. If it did, galaxies would fly apart. Recent calculations indicate that ordinary matter containing atoms makes up only 4 percent of the energy-matter content of the universe. “Dark energy” makes up 73 percent, and an unknown form of dark matter makes up the last 23 percent.
“It is often said that this is the ultimate Copernican Revolution,” said David Caldwell, a physicist at the University of California at Santa Barbara and chair of the CDMS Executive Committee. “Not only are we not at the center of the universe, but we are not even made of the same stuff as most of the universe.”
Measurements of the cosmic microwave background, residual radiation left over from the Big Bang, have recently placed severe constraints on the nature and amount of dark matter. The lightweight neutrino can account for only a few percent of the missing mass. If neutrinos constituted the main component of dark matter, they would act on the cosmic microwave background of the universe in ways that the recent Wilkinson Microwave Anisotropy Probe should have observed-but did not.
Meanwhile, particle physicists have kept a lookout for particles that will extend the Standard Model, the theory of fundamental particles and forces. Supersymmetry, a theory that takes a big step toward the unification of all of the forces of nature, predicts that every matter particle has a massive supersymmetric counterpart. No one has yet seen one of these “superpartners.” Theory specifies the neutralino as the lightest neutral superpartner, and the most stable, a necessary attribute for dark matter. The neutralino’s predicted abundance and rate of interaction also make it a likely dark matter candidate, and Caldwell noted the impact that CDMS II could have.
“Discovery,” he said, “would be a great breakthrough, one of the most important of the century.”
Only occasionally would a WIMP hit the nucleus of a terrestrial atom, and the constant background “noise” from more mundane particle events-such as the common cosmic rays constantly showering the earth-would normally drown out these rare interactions. Placing the CDMS II detector beneath 740 meters of earth screens out most particle noise from cosmic rays. Chilling the detector to 50 thousandths of a degree above absolute zero reduces background thermal energy to allow detection of individual particle collisions. Fermilab’s Bauer estimates that with sufficiently low backgrounds, CDMS needs only a few interactions to make a strong claim for detection of WIMPs.
“The powerful technology we deploy allows an unambiguous identification of events in the crystals caused by any new form of matter,” said CDMS cospokesperson Bernard Sadoulet of the University of California at Berkeley.
Cospokesperson Blas Cabrera of Stanford University concurred.
“We believe we have the best apparatus in the world in terms of being able to identify WIMPs,” Cabrera said.
“This endeavor is a good example of cooperation between the DOE’s Office of High Energy Physics and the National Science Foundation in helping scientists address the origin of the dark matter in the universe,” said Raymond Orbach, Director of the Department of Energy’s Office of Science.
“CDMS II is the kind of innovative and pathbreaking research NSF is proud to support,” said Michael Turner, Assistant Director for Math and Physical Sciences at the National Science Foundation. “If it detects a signal it may tell us what the dark matter is and give us an important clue as to how gravity fits together with the other forces. This type of experiment shows how the universe can be used as a laboratory for getting at the some of the most basic questions we can ask as well as how DOE and NSF are working together.”
While CDMS II watches for WIMPs, scientists at Fermilab’s Tevatron particle accelerator will try to create neutralinos by smashing protons and antiprotons together.
“CDMS can tell us the mass and interaction rate of the WIMP,” said collaborator Roger Dixon of Fermilab. “But it will take an accelerator to tell us whether it’s a neutralino.”
CDMS II collaborators include Brown University, Case Western Reserve University, Fermi National Accelerator Laboratory, Lawrence Berkeley National Accelerator Laboratory, National Institute of Standards and Technology, Princeton University, Santa Clara University, Stanford University, University of California at Berkeley, University of California at Santa Barbara, University of Colorado at Denver, University of Minnesota.
Funding for the CDMS II experiment comes from the Office of Science of the U.S. Department of Energy and the Astronomy and Physics Division of the National Science Foundation.
Fermilab is a national laboratory funded by the Office of Science of the U.S. Department of Energy and operated by Universities Research Association, Inc.
CDMS home page: http://cdms.berkeley.edu/index.html
- In these figures, the dotted red line divides events into those determined not to be WIMPs based on the relative timing of the heat to charge signals (left side) and those that could potentially be WIMPs based on that parameter (right side). The solid red box delineates the area of the graph in which WIMPs should occur based on both timing and the heat to charge ratio. Two events in separate detectors demonstrated the characteristics scientists predicted a WIMP would have. Credit: CDMS
- The curves dipping through this figure represent the results of several dark matter search experiments. The vertical scale represents the rate of WIMP scatters with nuclei while the horizontal scale is the mass of the WIMP. The gray line represents the 2008 results from the CDMS experiment. The blue line represents the most recent CDMS results. The solid black line represents the two results combined. The dotted black line represents the curve the combined results would have formed if CDMS had found no candidate events in 2009. The green and gray backgrounds represent areas that two theories of supersymmetry predict would contain dark matter. Credit: CDMS
- Dark matter detectors. The Cryogenic Dark Matter Search experiment uses five towers of six detectors each. Credit: Fermilab
- Dan Bauer, CDMS project manager and Fermilab scientist, removes one tower of detectors used in the Cryogenic Dark Matter Search experiment. Credit: Fermilab
- Closeup of a CDMS detector, made of crystal germanium. Credit: Fermilab
- The CDMS detectors, made of germanium, are located inside an ice box. A fridge provides the coolant to keep the detectors at close to absolute zero. Shielding material around the icebox minimizes the number of cosmic rays and other background particles that hit the detectors. Credit: CDMS collaboration
- The CDMS experiment has achieved the world’s most stringent limits on how often dark particles interact with ordinary matter and how heavy they are, in particular in the theoretically favored mass range of more than 40 times the proton mass (about 40 GeV/c2). The regions excluded are those above the solid lines. The black line is the most stringent limit. The bottom axis indicates the WIMP mass and the left axis refers to the frequency with which WIMPs interact with ordinary matter.
- Closeup of a detector in its mount. A detector of this kind, made of Silicon, was operated in the 1998 run. The photolithographically-fabricated thin film on the surface is the phonon sensor and represents a significant advance over the detectors used in the 1999 run. Silicon and germanium detectors, weighing 100 g and 250 g respectively, are used in CDMS II runs in the Soudan Mine.
- Project manager Dan Bauer from Fermilab holds one tower of detectors as Vuk Mandic, now at the University of Minnesota, examines them. Each tower of detectors contains 1 kilogram of germanium for detecting dark matter and 200 grams of silicon to distinguish WIMPs from neutrons. Thin layers of silicon, aluminum, and tungsten covering the detector surfaces measure both the heat and charge released when a particle interacts inside.
- A view of the inner layers of the cryostat with two towers installed. Detector towers are mounted in the holes covered by hexagonal plates. The coldest part of the cryostat stays at 10 mK (millikelvin, or thousandths of a degree above absolute zero) during operation. The surrounding layers are higher temperature stages of the cryostat. The cryostat is constructed using radiopure copper to provide a low-radioactivity environment for the extremely sensitive CDMS detectors.
- A scientist examines the shielding around the cryostat.
- The first detectors arrive at the Soudan Mine on February 21, 2003.
- A collaboration meeting at Soudan in March, 2003. The 12-institution collaboration includes 45 scientists.
- The Soudan Underground Mine was closed in 1963 and placed on the National Register of Historic Places in 1966. It is operated as a State Park by the Minnesota Department of Natural Resources, with 14 tours a day taking the historic elevator for a fast and clamorous ride nearly a half-mile below the surface. After descending, hard hat-wearing tourists can view old mine caverns with some of the equipment still standing in place. Since May 2002, tourists can also view the cavern housing the CDMS detector.
- Soudan is part of Minnesota’s Iron Range. Rich ore deposits were discovered in the area in 1865. Today, underground mines have largely given way to surface mining. The Soudan Underground Mine has served as a physics laboratory since 1979. The photo shows the view from the top of the tower above the Soudan Mine shaft.
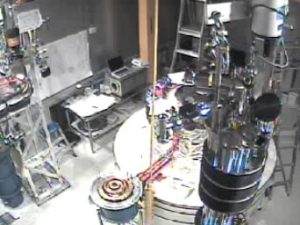
This video (1 min.) shows a time lapse of the construction of the CDMS experiment in 2003-2004. Watch video (QuickTime movie. May not work in all browsers.)
Credit: Priscilla Cushman
Background for the CDMS experiment
What does “setting limits” mean in an experiment?
Experimental physicists, such as those working on CDMS, frequently are faced with the situation of quantifying what it means when they have not yet found what they are searching for. Sometimes the result is that no events have been found, but more often it is the case that events have been seen which are consistent with expected sources of backgrounds. In either case, there are mathematical formulae for calculating “90% confidence level upper limits” on the rate of the signal which hasn’t been clearly detected. Such a limit implies that if you could do the same experiment 100 times, the result would be the same (or fewer) events detected in 90 of those experiments.
What is dark matter?
Judging by the way galaxies rotate, scientists have known for 70 years that the matter we can see does not provide enough gravitational pull to hold the galaxies together. There must exist some form of matter that does not emit or reflect light. According to the Wilkinson Microwave Anisotropy Probe, or WMAP, a survey of the microwave background radiation left over from the big bang, ordinary (“baryonic”) matter containing atoms makes up only 4% of the energy-matter contents in the Universe. “Dark energy” makes up 73%, and an unknown form of dark matter makes up the last 23%. (Most of the baryonic matter is dark matter too and resides in hydrogen and dust clouds and very dim clumps called massive compact halo objects. MACHOs include planets and cold dead stars like brown dwarfs and black holes.)
We can infer some of the properties of the nonbaryonic dark matter, such as its density, from the WMAP. The Sloan Digital Sky Survey has recently confirmed these results. We know that neutrinos, very light particles left over from the big bang in massive quantities, make up a small amount. WIMPs, or weakly interactive massive particles, may make up the rest.
What’s the difference between a neutrino and a neutralino?
Neither has an electric charge, and each probably makes up some of the missing matter but otherwise they have little in common. The neutrino carries almost no mass. It moves at nearly the speed of light, which makes it “hot dark matter.” This velocity means that it could not have made galaxies congeal in the early universe. Most neutrinos remain from the beginning of the universe, but particle decay and atomic fusion in stars continue to produce them.
Supersymmetry predicts the existence of the massive neutralino. We know it carries at least 46 times the mass of a proton because otherwise experiments at the Large Electron-Positron collider (LEP) at CERN would have detected their production. If it is a WIMP, it travels through the universe at 1/1000 the speed of light, making it “cold dark matter.” Neutralinos were produced at the beginning of the universe but exist in fewer numbers than the neutrino because their great mass makes them harder to produce, and they annihilate each other. They both pass through the Earth in large quantities.
What is a WIMP?
Weakly interactive massive particles may make up most of the dark matter, if they have a mass of 10 to 10000 times the mass of the proton. They only interact via the weak force and gravity (not the strong or electromagnetic force), so they only disturb atoms when they collide with a nucleus. Atoms contain mostly empty space, so this rarely happens. Photons pass right through them. As many as 10 trillion WIMPs should pass through one kilogram of the Earth in a second but perhaps as few as one per day will interact.
What is supersymmetry?
The Standard Model describes all of the particles and forces in the universe, but it does not adequately explain the origin of mass. To solve this problem, in 1982 some theorists proposed an extension to the Standard Model where every mass particle (the quark, electron, etc) and every force-carrying particle (the photon, graviton, etc.) has an associated “superpartner” that differs only in its spin and mass. The undetected superpartners are much more massive than the particles observed so far. The lightest neutral supersymmetric particle is the neutralino. With an expected mass of 50-1000 billion electron volts (GeV)-a proton’s mass is 1 GeV-and weak interaction with the baryons (protons and neutrons) that make up everyday matter, they are considered WIMPs.
Why are the detectors underground?
Cosmic rays hit the surface of the Earth and the reactions produce neutrons. Placing the detectors deep underground shields them from most of the cosmic rays that would produce neutrons.
Why are the detectors so cold?
The cryostat uses six nested layers to cool the detectors to 50 millikelvin (thousandths of a degree above absolute zero.) This reduces the background vibrations of the detector’s atoms and makes them more sensitive to individual particle collisions.
How do the detectors work?
The experimental set-up for CDMS II contains two towers of detectors. Each tower contains a kilogram of germanium for detecting dark matter and 200 grams of silicon to distinguish WIMPs from neutrons. Supersymmetry models predict that only a few WIMPs per year, one per day at most, will interact with the detectors. The biggest challenge involves sorting them from background interactions due to electrons, neutrons, and gamma rays.
When a WIMP hits a germanium nucleus, the nucleus recoils and vibrates the whole germanium crystal. This warms the thin aluminum and tungsten outer layers, which an electrical circuit measures. Photons and electrons, however, strike the germanium’s electrons. A charge collection plate measures ionization resulting from this type of collision and uses it to separate these interactions from those of WIMPs. The ratio of charge to heat for each event tells whether a particle struck the nucleus, as WIMPs do, or simply rattled the electrons surrounding the nucleus, as most background particles do.
Incoming neutrons also strike the germanium nucleus, so they more closely resemble WIMPs. The germanium detectors sit in a stack with detectors made of silicon. A silicon atom has a smaller nucleus, and so will be hit less frequently by WIMPs. The strong nuclear force does not affect WIMPs, but it does affect neutrons and so neutrons will hit nuclei of different sizes at about the same rate. A higher collision rate in the germanium than the silicon will indicate the interaction of WIMPs.
Does CDMS have any competition?
CDMS has several competitors around the world. DAMA, a collaboration between Italian and Chinese scientists in the Gran Sasso tunnel in Italy, has collected data over the past six years and shows seasonal changes in events. They argue that this modulation results from the Earth traveling with or against the flow of cosmic dark matter particles and provides direct evidence for WIMPs. The scientists have addressed concerns that other factors–including temperature, humidity, or radon–may account for the data, but according to Bauer, “they don’t address all of the conventional explanations at the level of detail that I think an extraordinary claim like that requires.”
The closest competition comes from a French project called EDELWEISS, which also uses a germanium detector and measures heat and ionization. They have collecting data for three years, but they have fewer detectors than CDMS right now and they have less-sophisticated background rejection capability.