CDMS II presents new results on Weakly Interacting Massive Particles that could make up most of the matter of our universe
BATAVIA, Ill.- With the first data from their underground observatory in Northern Minnesota, scientists of the Cryogenic Dark Matter Search have peered with greater sensitivity than ever before into the suspected realm of the WIMPs. The sighting of weakly interacting massive particles could solve the double mystery of dark matter on the cosmic scale and of supersymmetry on the subatomic scale.
The CDMS II result, described in a paper submitted to Physical Review Letters, shows with 90 percent certainty that the interaction rate of a WIMP with mass 60 GeV must be less than 4 x 10-43 cm2 or about one interaction every 25 days per kilogram of germanium, the material in the experiment’s detector. This result tells researchers more than they have ever known before about WIMPS, if they exist. The measurements from the CDMS II detectors are at least four times more sensitive than the best previous measurement offered by the EDELWEISS experiment, an underground European experiment near Grenoble, France.
“Think of this improved sensitivity like a new telescope with twice the diameter and thus four times the light collection of any that came before it,” said CDMS II cospokesperson Blas Cabrera of Stanford University. “We are now able to look for a signal that is just one-fourth as bright as any we have seen before. Over the next few years, we expect to improve our sensitivity by a factor of 20 or more.”
The results are being presented at the April Meeting of the American Physical Society on May 3 and 4 in Denver by Harry Nelson and graduate student Joel Sanders, both of the University of California-Santa Barbara, and by Gensheng Wang and Sharmila Kamat of Case Western Reserve University.
“We know that neither our Standard Model of particle physics nor our model of the cosmos is complete,” said CDMS II spokesperson Bernard Sadoulet of the University of California at Berkeley. “This particular missing piece seems to fit both puzzles. We are seeing the same shape from two different directions.”
WIMPs, which carry no charge, are a study in contradictions. While physicists expect them to have about 100 times the mass of protons, their ghostly nature allows them to slip through ordinary matter leaving barely a trace. The term “weakly interacting” refers not to the amount of energy deposited when they interact with normal matter, but rather to the fact that they interact extremely infrequently. In fact, as many as a hundred billion WIMPs may have streamed through your body as you read these first few sentences.
With 48 scientists from 13 institutions, plus another 28 engineering, technical and administrative staffers, CDMS II operates with funding from the Office of Science of the U.S. Department of Energy, from the Astronomy and Physics Divisions of the National Science Foundation and from member institutions. The DOE’s Fermi National Accelerator Laboratory provides the project management for CDMS II.
“The nature of dark matter is fundamental to our understanding of the formation and evolution of the universe,” said Dr. Raymond L. Orbach, Director of DOE’s Office of Science. “This experiment could not have succeeded without the active collaboration of the DOE’s Office of Science and the National Science Foundation.”
Michael Turner, Assistant Director for Math and Physical Sciences at NSF, described identifying the constituent of the dark matter as one of the great challenges in both astrophysics and particle physics.
“Dark matter holds together all structures in the universe-including our own Milky Way-and we still do not know what the dark matter is made of,” Turner said. “The working hypothesis is that it is a new form of matter-which, if correct will shed light on the inner workings of the elementary forces and particles. In pursuing the solution to this important puzzle, CDMS is now at the head of the pack, with another factor of 20 in sensitivity still to come.”
Dark matter in the universe is detected through its gravitational effects on all cosmic scales, from the growth of structure in the early universe to the stability of galaxies today. Cosmological data from many sources confirm that this unseen dark matter totals more than seven times the amount of ordinary visible matter forming the stars, planets and other objects in the universe.
“Something out there formed the galaxies and holds them together today, and it neither emits nor absorbs light,” said Cabrera. “The mass of the stars in a galaxy is only 10 percent of the mass of the entire galaxy, so the stars are like Christmas tree lights decorating the living room of a large dark house.”
Physicists also believe WIMPs could be the as-yet unobserved subatomic particles called neutralinos. These would be evidence for the theory of supersymmetry, introducing intriguing new physics beyond today’s Standard Model of fundamental particles and forces.
Supersymmetry predicts that every known particle has a supersymmetric partner with complementary properties, although none of these partners has yet been observed. However, many models of supersymmetry predict that the lightest supersymmetric particle, called the neutralino, has a mass about 100 times that of the proton.
“Theorists came up with all of these so-called ‘supersymmetric partners’ of the known particles to explain problems on the tiniest distance scales,” said Dan Akerib of Case Western Reserve University. “In one of those fascinating connections of the very large and the very small, the lightest of these superpartners could be the missing piece of the puzzle for explaining what we observe on the very largest distance scales.”
The CDMS II team practices “underground astronomy,” with particle detectors located nearly a half-mile below the earth’s surface in a former iron mine in Soudan, Minnesota. The 2,341 feet of the earth’s crust shields out cosmic rays and the background particles they produce. The detectors are made of germanium and silicon, semiconductor crystals with similar properties. The detectors are chilled to within one-tenth of a degree of absolute zero, so cold that molecular motion becomes negligible. The detectors simultaneously measure the charge and vibration produced by particle interactions within the crystals. WIMPS will signal their presence by releasing less charge than other particles for the same amount of vibration.
“Our detectors act like a telescope equipped with filters that allow astronomers to distinguish one color of light from another,” said CDMS II project manager Dan Bauer of Fermilab. “Only, in our case, we are trying to filter out conventional particles in favor of dark matter WIMPS.”
Physicist Earl Peterson of the University Minnesota oversees the Soudan Underground Laboratory, also home to Fermilab’s long-baseline neutrino experiment, the Main Injector Neutrino Oscillation Search.
“I’m excited about the significant new result from CDMS II, and I congratulate the collaboration,” Peterson said. “I’m pleased that the facilities of the Soudan Laboratory contributed to the success of CDMS II. And I’m especially pleased that the work of Fermilab and the University of Minnesota in expanding the Soudan Laboratory has resulted in superb new physics.”
As CDSMII searches for WIMPs over the next few years, either the dark matter of our universe will be discovered, or a large range of supersymmetric models will be excluded from possibility. Either way, the CDMS II experiment will play a major role in advancing our understanding of particle physics and of the cosmos.
The CDMS II collaborating institutions include Brown University, Case Western Reserve University, Fermi National Accelerator Laboratory, Lawrence Berkeley National Laboratory, the National Institutes of Standards and Technology, Princeton University, Santa Clara University, Stanford University, the University of California-Berkeley, the University of California-Santa Barbara, the University of Colorado at Denver, the University of Florida, and the University of Minnesota.
Fermilab is a DOE Office of Science national laboratory operated under contract by Universities Research Association, Inc.
CDMS home page:
http://cdms.berkeley.edu/index.html
- In these figures, the dotted red line divides events into those determined not to be WIMPs based on the relative timing of the heat to charge signals (left side) and those that could potentially be WIMPs based on that parameter (right side). The solid red box delineates the area of the graph in which WIMPs should occur based on both timing and the heat to charge ratio. Two events in separate detectors demonstrated the characteristics scientists predicted a WIMP would have. Credit: CDMS
- The curves dipping through this figure represent the results of several dark matter search experiments. The vertical scale represents the rate of WIMP scatters with nuclei while the horizontal scale is the mass of the WIMP. The gray line represents the 2008 results from the CDMS experiment. The blue line represents the most recent CDMS results. The solid black line represents the two results combined. The dotted black line represents the curve the combined results would have formed if CDMS had found no candidate events in 2009. The green and gray backgrounds represent areas that two theories of supersymmetry predict would contain dark matter. Credit: CDMS
- Dark matter detectors. The Cryogenic Dark Matter Search experiment uses five towers of six detectors each. Credit: Fermilab
- Dan Bauer, CDMS project manager and Fermilab scientist, removes one tower of detectors used in the Cryogenic Dark Matter Search experiment. Credit: Fermilab
- Closeup of a CDMS detector, made of crystal germanium. Credit: Fermilab
- The CDMS detectors, made of germanium, are located inside an ice box. A fridge provides the coolant to keep the detectors at close to absolute zero. Shielding material around the icebox minimizes the number of cosmic rays and other background particles that hit the detectors. Credit: CDMS collaboration
- The CDMS experiment has achieved the world’s most stringent limits on how often dark particles interact with ordinary matter and how heavy they are, in particular in the theoretically favored mass range of more than 40 times the proton mass (about 40 GeV/c2). The regions excluded are those above the solid lines. The black line is the most stringent limit. The bottom axis indicates the WIMP mass and the left axis refers to the frequency with which WIMPs interact with ordinary matter.
- Closeup of a detector in its mount. A detector of this kind, made of Silicon, was operated in the 1998 run. The photolithographically-fabricated thin film on the surface is the phonon sensor and represents a significant advance over the detectors used in the 1999 run. Silicon and germanium detectors, weighing 100 g and 250 g respectively, are used in CDMS II runs in the Soudan Mine.
- Project manager Dan Bauer from Fermilab holds one tower of detectors as Vuk Mandic, now at the University of Minnesota, examines them. Each tower of detectors contains 1 kilogram of germanium for detecting dark matter and 200 grams of silicon to distinguish WIMPs from neutrons. Thin layers of silicon, aluminum, and tungsten covering the detector surfaces measure both the heat and charge released when a particle interacts inside.
- A view of the inner layers of the cryostat with two towers installed. Detector towers are mounted in the holes covered by hexagonal plates. The coldest part of the cryostat stays at 10 mK (millikelvin, or thousandths of a degree above absolute zero) during operation. The surrounding layers are higher temperature stages of the cryostat. The cryostat is constructed using radiopure copper to provide a low-radioactivity environment for the extremely sensitive CDMS detectors.
- A scientist examines the shielding around the cryostat.
- The first detectors arrive at the Soudan Mine on February 21, 2003.
- A collaboration meeting at Soudan in March, 2003. The 12-institution collaboration includes 45 scientists.
- The Soudan Underground Mine was closed in 1963 and placed on the National Register of Historic Places in 1966. It is operated as a State Park by the Minnesota Department of Natural Resources, with 14 tours a day taking the historic elevator for a fast and clamorous ride nearly a half-mile below the surface. After descending, hard hat-wearing tourists can view old mine caverns with some of the equipment still standing in place. Since May 2002, tourists can also view the cavern housing the CDMS detector.
- Soudan is part of Minnesota’s Iron Range. Rich ore deposits were discovered in the area in 1865. Today, underground mines have largely given way to surface mining. The Soudan Underground Mine has served as a physics laboratory since 1979. The photo shows the view from the top of the tower above the Soudan Mine shaft.
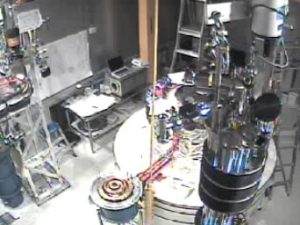
This video (1 min.) shows a time lapse of the construction of the CDMS experiment in 2003-2004. Watch video (QuickTime movie. May not work in all browsers.)
Credit: Priscilla Cushman
Background for the CDMS experiment
What does “setting limits” mean in an experiment?
Experimental physicists, such as those working on CDMS, frequently are faced with the situation of quantifying what it means when they have not yet found what they are searching for. Sometimes the result is that no events have been found, but more often it is the case that events have been seen which are consistent with expected sources of backgrounds. In either case, there are mathematical formulae for calculating “90% confidence level upper limits” on the rate of the signal which hasn’t been clearly detected. Such a limit implies that if you could do the same experiment 100 times, the result would be the same (or fewer) events detected in 90 of those experiments.
What is dark matter?
Judging by the way galaxies rotate, scientists have known for 70 years that the matter we can see does not provide enough gravitational pull to hold the galaxies together. There must exist some form of matter that does not emit or reflect light. According to the Wilkinson Microwave Anisotropy Probe, or WMAP, a survey of the microwave background radiation left over from the big bang, ordinary (“baryonic”) matter containing atoms makes up only 4% of the energy-matter contents in the Universe. “Dark energy” makes up 73%, and an unknown form of dark matter makes up the last 23%. (Most of the baryonic matter is dark matter too and resides in hydrogen and dust clouds and very dim clumps called massive compact halo objects. MACHOs include planets and cold dead stars like brown dwarfs and black holes.)
We can infer some of the properties of the nonbaryonic dark matter, such as its density, from the WMAP. The Sloan Digital Sky Survey has recently confirmed these results. We know that neutrinos, very light particles left over from the big bang in massive quantities, make up a small amount. WIMPs, or weakly interactive massive particles, may make up the rest.
What’s the difference between a neutrino and a neutralino?
Neither has an electric charge, and each probably makes up some of the missing matter but otherwise they have little in common. The neutrino carries almost no mass. It moves at nearly the speed of light, which makes it “hot dark matter.” This velocity means that it could not have made galaxies congeal in the early universe. Most neutrinos remain from the beginning of the universe, but particle decay and atomic fusion in stars continue to produce them.
Supersymmetry predicts the existence of the massive neutralino. We know it carries at least 46 times the mass of a proton because otherwise experiments at the Large Electron-Positron collider (LEP) at CERN would have detected their production. If it is a WIMP, it travels through the universe at 1/1000 the speed of light, making it “cold dark matter.” Neutralinos were produced at the beginning of the universe but exist in fewer numbers than the neutrino because their great mass makes them harder to produce, and they annihilate each other. They both pass through the Earth in large quantities.
What is a WIMP?
Weakly interactive massive particles may make up most of the dark matter, if they have a mass of 10 to 10000 times the mass of the proton. They only interact via the weak force and gravity (not the strong or electromagnetic force), so they only disturb atoms when they collide with a nucleus. Atoms contain mostly empty space, so this rarely happens. Photons pass right through them. As many as 10 trillion WIMPs should pass through one kilogram of the Earth in a second but perhaps as few as one per day will interact.
What is supersymmetry?
The Standard Model describes all of the particles and forces in the universe, but it does not adequately explain the origin of mass. To solve this problem, in 1982 some theorists proposed an extension to the Standard Model where every mass particle (the quark, electron, etc) and every force-carrying particle (the photon, graviton, etc.) has an associated “superpartner” that differs only in its spin and mass. The undetected superpartners are much more massive than the particles observed so far. The lightest neutral supersymmetric particle is the neutralino. With an expected mass of 50-1000 billion electron volts (GeV)-a proton’s mass is 1 GeV-and weak interaction with the baryons (protons and neutrons) that make up everyday matter, they are considered WIMPs.
Why are the detectors underground?
Cosmic rays hit the surface of the Earth and the reactions produce neutrons. Placing the detectors deep underground shields them from most of the cosmic rays that would produce neutrons.
Why are the detectors so cold?
The cryostat uses six nested layers to cool the detectors to 50 millikelvin (thousandths of a degree above absolute zero.) This reduces the background vibrations of the detector’s atoms and makes them more sensitive to individual particle collisions.
How do the detectors work?
The experimental set-up for CDMS II contains two towers of detectors. Each tower contains a kilogram of germanium for detecting dark matter and 200 grams of silicon to distinguish WIMPs from neutrons. Supersymmetry models predict that only a few WIMPs per year, one per day at most, will interact with the detectors. The biggest challenge involves sorting them from background interactions due to electrons, neutrons, and gamma rays.
When a WIMP hits a germanium nucleus, the nucleus recoils and vibrates the whole germanium crystal. This warms the thin aluminum and tungsten outer layers, which an electrical circuit measures. Photons and electrons, however, strike the germanium’s electrons. A charge collection plate measures ionization resulting from this type of collision and uses it to separate these interactions from those of WIMPs. The ratio of charge to heat for each event tells whether a particle struck the nucleus, as WIMPs do, or simply rattled the electrons surrounding the nucleus, as most background particles do.
Incoming neutrons also strike the germanium nucleus, so they more closely resemble WIMPs. The germanium detectors sit in a stack with detectors made of silicon. A silicon atom has a smaller nucleus, and so will be hit less frequently by WIMPs. The strong nuclear force does not affect WIMPs, but it does affect neutrons and so neutrons will hit nuclei of different sizes at about the same rate. A higher collision rate in the germanium than the silicon will indicate the interaction of WIMPs.
Does CDMS have any competition?
CDMS has several competitors around the world. DAMA, a collaboration between Italian and Chinese scientists in the Gran Sasso tunnel in Italy, has collected data over the past six years and shows seasonal changes in events. They argue that this modulation results from the Earth traveling with or against the flow of cosmic dark matter particles and provides direct evidence for WIMPs. The scientists have addressed concerns that other factors–including temperature, humidity, or radon–may account for the data, but according to Bauer, “they don’t address all of the conventional explanations at the level of detail that I think an extraordinary claim like that requires.”
The closest competition comes from a French project called EDELWEISS, which also uses a germanium detector and measures heat and ionization. They have collecting data for three years, but they have fewer detectors than CDMS right now and they have less-sophisticated background rejection capability.