Superconducting radio-frequency (SRF) cavities are the “muscle” of many modern particle accelerators. By cooling these devices to cryogenic temperatures (usually around 2 Kelvin, or minus 456 degrees Fahrenheit) and inputting electric power, SRF cavities increase the energy of beams of charged particles passing through them. Making cavities out of superconducting materials dramatically increases their efficiency (represented by a cavity’s quality factor, or Q), allowing them to accelerate beams to high energies over short distances, without leaving long cool-down times between particle beam pulses. Researchers are developing new SRF materials with the potential to operate at higher temperatures — in the 4-5 K range — in order to ease the requirements for cooling. In a new experiment using films of the superconductor Nb3Sn, or niobium-tin, experimenters at Fermilab showed record performance for a low frequency (less than 1 gigahertz) SRF cavity.
In the Fermilab SRF cavity program, scientists and engineers apply a thin film of niobium-tin to the inside of niobium accelerating cavities to improve their efficiency. The program builds on pioneering work in niobium-tin cavities performed in the past. This includes a study at CERN from the 1980s, one of the very few experiments on niobium-tin cavities at lower frequency, in the sub-GHz range.
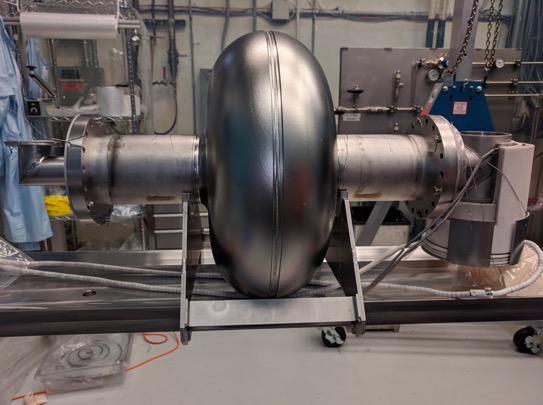
A low-frequency, single-cell cavity is under preparation niobium-tin coating. Photo courtesy of Sam Posen
Low-frequency cavities have certain advantages, including cryogenic efficiency, the ability to accept larger beam sizes, and the ability to accelerate particles in a wide range of velocities below the speed of light. On the other hand, at lower frequencies, cavities tend to be larger, meaning more surface area to coat with niobium-tin, which can make the coating process more challenging.
Our Fermilab SRF group coated a 650-MHz single-cell PIP-II-style cavity, our first attempt to coat a low frequency cavity. We achieved record performance for a cavity of this type. Crucially, the cavity shows very high quality factor at temperatures in the range of 4-5 K, about 10 times higher than before coating, when it was a standard niobium cavity.
This performance has major implications for a program to develop a compact SRF accelerator at the Illinois Accelerator Research Center (IARC). The compact SRF accelerator being developed by IARC takes advantage of the high critical temperature of niobium-tin to allow the accelerator to operate in the 4-5 K range, where it is possible to cool the cavity by a cryocooler.
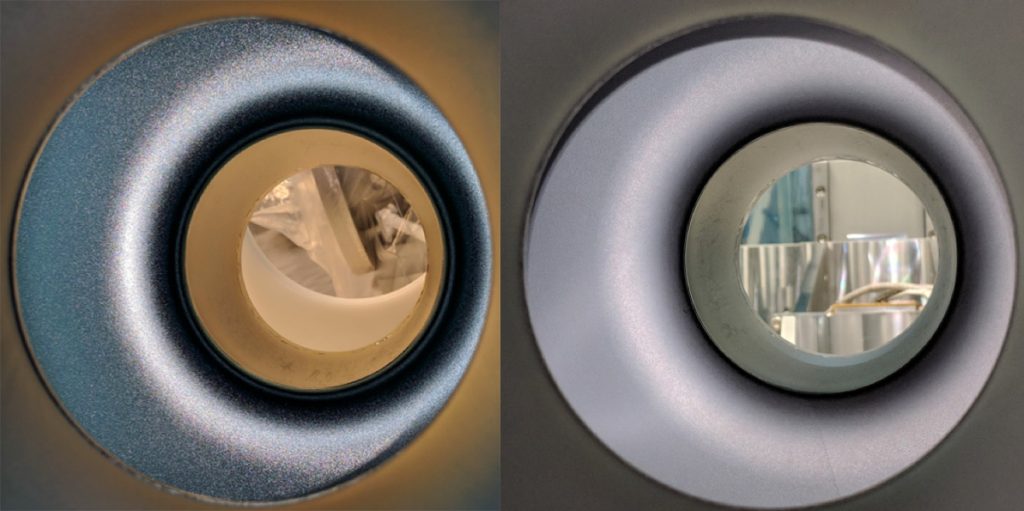
Interior cavity surface is shown before coating (left), showing the niobium surface, and after coating (right), showing the niobium-tin surface. Photos courtesy of Sam Posen
Unlike a liquid-helium cryogenic plant, a cryocooler is a low-cost, small footprint, low-maintenance, turn-key cooling system. This could be a game changer for small-scale accelerators. It could make new applications feasible, particularly for stand-alone systems where a connection to a cryogenic plant would not be practical. IARC’s grant from ERDC includes support for the development of niobium-tin cavities for use in the compact accelerator.
In this experiment, a cavity of the frequency appropriate for the compact accelerator was coated with niobium-tin and tested. At the accelerating field specification, the cavity showed quality factor exceeding the requirements, a major milestone for the IARC program.
While these results show promise for small scale accelerator applications, with additional development, niobium-tin cavities have the potential to improve large-scale accelerators as well. For a given heat load, operating a large cryogenic plant in the 4-5 K range offers a substantial savings in both infrastructure and operating costs compared to 2 K, where most niobium-based SRF accelerators operate. If we the performance of niobium-tin cavities can be pushed closer to the predicted ultimate potential of the material, it could open new opportunities in applications ranging from high-energy physics to X-ray science.
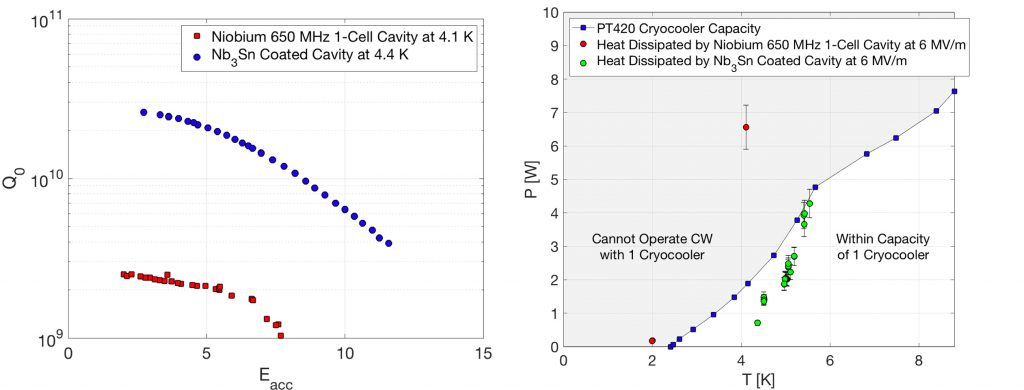
The plot on the left compares the 650-MHz cavity’s 4-5 K performance before (red) and after (blue) coating with niobium-tin. The plot on the right shows the heat dissipation compared to cryocooler capacity as a function of temperature.
This is a result we’re very proud of and an important milestone. Compared to niobium, which has been under intense development for 50 years, niobium-tin is a relatively immature technology, but it’s already showing the potential to enable new applications.
Fermilab’s Nb3Sn SRF cavity program began in 2015, with support for different aspects of the program coming from Fermilab Laboratory Directed Research and Development, a DOE Early Career Award, and a grant from the U.S. Army Engineer Research and Development Center. The goals of the program are to push the performance of niobium-tin SRF cavities and to scale up from small R&D cavities to full-scale accelerator-type structures. Our SRF group collaborates with Northwestern University’s Materials Science and Engineering Department to analyze our niobium-tin films (even down to the subnanometer scale) through the Center for Applied Physics and Superconducting Technology.
Sam Posen is the deputy head of the SRF Measurements and Research Department and a recipient of a 2016 DOE Early Career Award, which supports this work on developing superconducting films for particle accelerators.