How do you detect a particle that has almost no mass, feels only two of the four fundamental forces, and can travel unhindered through solid lead for an entire light-year without ever interacting with matter? This is the problem posed by neutrinos, ghostly particles that are generated in the trillions by nuclear reactions in stars, including our sun, and on Earth. Scientists can also produce neutrinos to study in controlled experiments using particle accelerators. One of the ways neutrinos can be detected is with large vats filled with liquid argon and wrapped with a complex web of integrated circuitry that can operate in temperatures colder than the average day on Neptune.
Industry does not typically use electronics that operate at cryogenic temperatures, so particle physicists have had to engineer their own. A collaboration of several Department of Energy national labs, including Fermilab, has been developing prototypes of the electronics that will ultimately be used in the international Deep Underground Neutrino Experiment, called DUNE, hosted by Fermilab. DUNE will generate an intense beam of neutrinos at Fermilab in Illinois and send it 800 miles through the Earth’s crust to detectors in South Dakota. Results from the experiment may help scientists understand why there is more matter than antimatter, an imbalance that led to the formation of our universe.
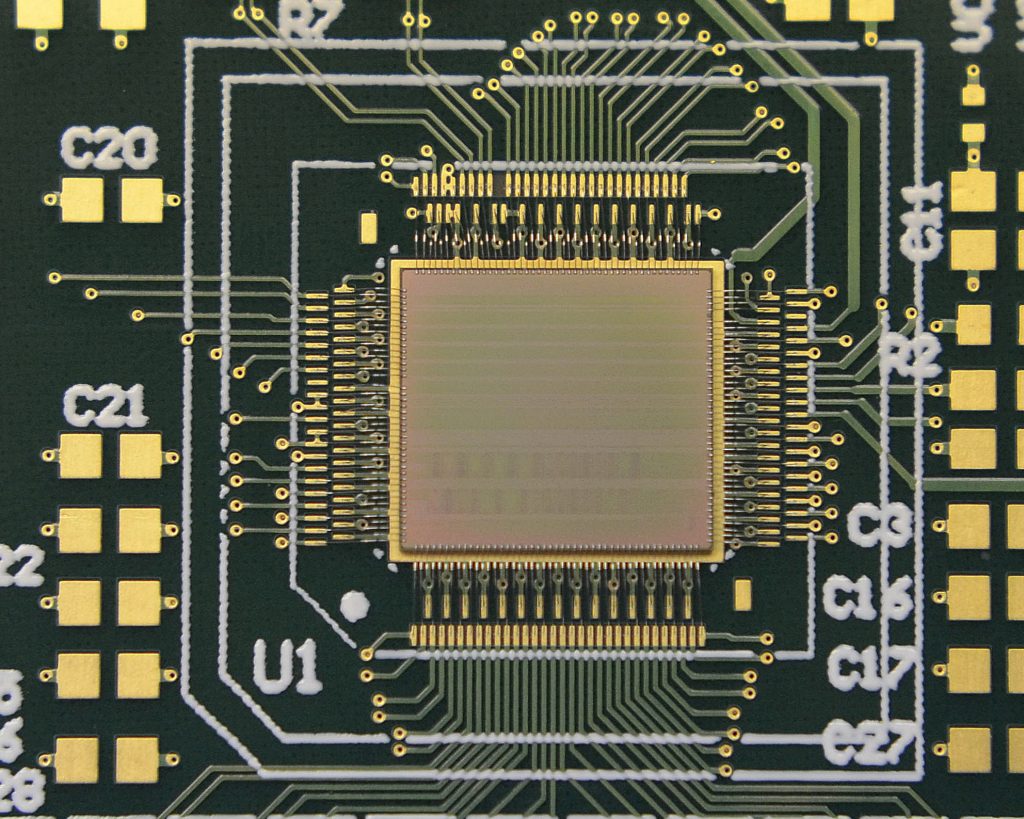
Analog-to-digital convertors built to work at cryogenic temperatures, such as the prototype pictured here, will operate inside of liquid-argon chambers in the Deep Underground Neutrino Experiment. Photo: Alber Dyer, Fermilab
Physics and chill
DUNE’s neutrino detectors will be massive: a total of four tanks, each as high as a four-story building, will contain a combined 70,000 tons of liquid argon and be situated in a cavern a mile beneath Earth’s surface.
Argon occurs naturally as a gas in our atmosphere, and turning it into a liquid entails chilling it to extremely cold temperatures. The atomic nuclei of liquid argon are so densely packed together that some of the famously elusive neutrinos traveling from Fermilab will interact with them, leaving behind tell-tale signs of their passing. The resulting collision produces different particles that scatter in all directions, including electrons, which physicists use to reconstruct the path of the otherwise invisible neutrino.
A strong electric field maintained within the detector causes the free electrons to drift toward wires attached to sensitive electronics. As the electrons travel past the wires, they generate small voltage pulses that are recorded by electronics in the liquid-argon chamber. Amplifiers in the chamber then boost the signal by increasing the voltage, after which they are converted to digital data. Finally, the signals collected and digitized across the entire chamber are merged together and sent to computers outside the detector for storage and analysis.
Challenges for chilled electronics
The electronics in neutrino detectors work the same way as the technology we use in our everyday lives, with one major exception. The integrated circuitry in our phones, computers, cameras, cars, microwaves and other devices has been developed to operate at or around room temperature, down to about minus 40 degrees Celsius. The liquid argon in neutrino detectors, however, is cooled to around minus 200 degrees.
“If you use electronics designed to work at room temperature, rarely do you find that they work anywhere nearly as well as those designed to operate at cryogenic temperatures,” said Fermilab scientist David Christian.
In the past, this issue was sidestepped altogether by placing the electronic circuitry outside of the argon tanks. But when you’re measuring a limited number of electrons, even the slightest amount of electronics noise can mask the signal you’re looking for.
The easiest way to mitigate the problem involves the same tactic you use to keep food from spoiling: Keep it cold. If all the electronics are submerged in the liquid argon, there are fewer thermal vibrations from atoms and a larger signal-to-noise ratio. Placing the electronics in the liquid-argon tank has the added benefit of decreasing the amount of wire you have to use to deliver signals to the amplifiers. If, for example, amplifiers and analog-to-digital converters are kept outside the chamber (as they are in some neutrino detectors), long wires have to connect them to the detectors on the inside.
“If you put the electronics inside the cold chamber, you have much shorter wires and therefore lower noise,” said Carl Grace, an engineer at Lawrence Berkeley National Laboratory. “You amplify the signal and digitize it in the argon chamber. You then have a digital interface to the outside world in which noise is no longer a concern.”
There are several design challenges these teams have had to overcome during development, not the least of which was determining how to test the durability of the devices.
“These chips will have to operate for a minimum of 20-odd years, hopefully longer,” Grace said. “And because of the nature of the argon chambers, the electronics that get put inside of them can’t cannot be changed. They cannot be swapped out or repaired in any way.”
Since Grace and his team don’t have 20 years in which to test their prototypes, they’ve approximated the effects of aging by increasing the amount of voltage powering the chips to simulate the wear and tear of regular, long-term operation.
“We take the electronics, cool them down and then elevate their voltage to accelerate their aging,” Grace said. “By observing their behavior over a relatively short period of time, we can we can then estimate how long the electronics would last if they were operated at the voltages for which they were designed.”
Resistance in circuits
Not only do these circuits need to be built to last for decades, they also need to be made more durable in another way.
Electronic circuitry has a certain amount of resistance to the electric current flowing through it. As electrons pass through a circuit, they interact with the vibrating atoms within the conducting material, which slows them down. But these interactions are reduced when the electronics are cooled to cryogenic temperatures, and the electrons that constitute the signal move more quickly on average.
This is a good thing in terms of output; the integrated circuits being built for DUNE will work more efficiently when placed in the liquid argon. But, as the electrons travel faster through the circuits as temperatures drop, they can begin to do damage to the circuitry itself.
“If electrons have a high enough kinetic energy, they can actually start ripping atoms from the crystal structure of the conducting material,” Grace said. “It’s like bullets hitting a wall. The wall starts to lose integrity over time.”
DUNE chips are designed to mitigate this effect. The chips are fabricated using large constituent devices to minimize the amount of damage accrued, and they are used at lower voltages than normally used at room temperature. Scientists can also adjust operating parameters over time to compensate for any damage that occurs during their many years of use.
Timeline to completion
With preparations for the DUNE well underway and the experiment slated to begin generating data by 2027, scientists from many institutions have been hard at work developing electronic prototypes.
Scientists at Brookhaven National Laboratory are working on perfecting the amplifier, while teams from Fermilab, Brookhaven and Berkeley labs are collaborating on the analog-to-digital converter design. Fermilab has also teamed up with Southern Methodist University to develop the electronic component that merges all of the data within an argon tank before it’s transmitted to electronics located outside the cold detector. Finally, researchers working on a competing design at SLAC National Accelerator Laboratory are trying to find a way to efficiently combine all three components into one integrated circuit.
The various teams plan to submit their circuit designs this summer for review. The selected designs will be built and ultimately installed in the DUNE neutrino detectors at the Sanford Underground Neutrino Facility in South Dakota.
U.S. work on LBNF/DUNE is supported by the Department of Energy Office of Science.
Fermilab is supported by the Office of Science of the U.S. Department of Energy. The Office of Science is the single largest supporter of basic research in the physical sciences in the United States and is working to address some of the most pressing challenges of our time. For more information, visit science.energy.gov.