A 100-meter-deep shaft at Fermilab — constructed for a neutrino experiment many years ago — will become home to a new quantum experiment that will explore the nature of dark matter and gravitational waves.
Scientists of the MAGIS-100 collaboration will drop groups of atoms down a vacuum tube, followed by laser light, to explore aspects of quantum physics. The experiment aims to reveal the presence of ultralight dark matter particles and pave the way for detecting gravitational waves of lower frequency than previously detected.
According to the laws of quantum physics, atoms can be in two places at once: a superposition of two quantum states. Similar to a wave breaking around a rock, an atom can form two wave crests at different locations, which then recombine.
The 100-meter-long Matter-wave Atomic Gradiometer Interferometric Sensor – nicknamed MAGIS-100 – will be the world’s largest atom interferometer and push the boundaries of how far an atom can be driven apart from itself. One of the better known interferometers is LIGO, funded by the National Science Foundation. LIGO is used to detect gravitational waves by splitting a light wave in half and sending the light to two equidistant detectors. Any difference in arrival time means a gravity wave has passed through and distorted space.
As its name suggests, an atom interferometer measures that difference, but for atomic waves.
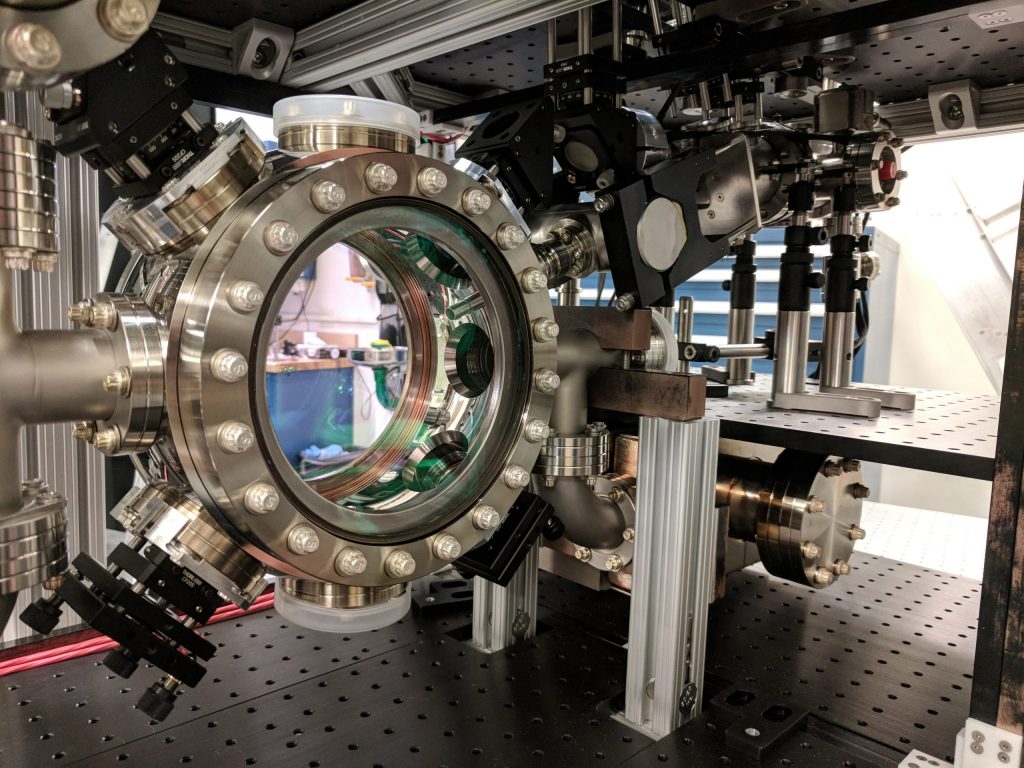
MAGIS-100 will push the boundaries of quantum physics into macroscopic scales, providing a gateway for dark matter searches and tests of gravitational waves. Photo: Stanford University
“We’ll be manipulating atoms on a human scale, achieving separations of about the height of a person,” explained Fermilab scientist Roni Harnik. “That has never been done before.”
Light from a laser will travel from one end of the vacuum tube to the other and back, illuminating clouds of falling atoms to measure the different atomic phases that result from a particle of light interacting with a single atom.
This technique, called atom interferometry, typically has used rubidium atoms. But new advances by the MAGIS group at Stanford University make it possible to use strontium atoms instead.
This change means that, when the laser interacts with an electron within a strontium atom, the electron will be energized for a longer amount of time – on the order of minutes as contrasted with rubidium’s nanoseconds. This increased lifetime makes strontium a great atomic clock and ideal for measuring the amount of time it takes the light from the laser to repeatedly traverse the length of the vacuum tube.
Combined with Fermilab’s expertise in engineering particle accelerators and other large scientific instruments, these technological advances make it possible to construct this interferometer and broach new realms.
Pushing the quantum boundaries
In an effort to illustrate the principles of quantum mechanics, Erwin Schrödinger devised his famous thought experiment commonly referred to as Schrödinger’s cat. The basic idea: If you placed a cat in a sealed box with a vial of randomly activated poison, then, until the box is opened, a common interpretation is that quantum mechanics says the cat can be considered both alive and dead.
It’s a good example of what physicists mean when they say that atoms can be in two quantum states at once, or in superposition.
Atomic clocks rely on this kind of superposition of a single atom to reach the level of precision that they are capable of.
MAGIS-100 will push the boundaries of how far two different states of an atom can be driven apart. Currently, Stanford University holds the record at 54 centimeters. MAGIS-100 anticipates that they will be able to at least triple that distance.
In addition to increasing the distance, MAGIS-100 also hopes to increase the amount of time an atom spends in superposition. The longest recorded separation for freely falling atoms is currently clocked in at around two seconds, but MAGIS-100 aims to push that to somewhere between five and 10 seconds.
Searching for elusive particles
The gravitational effects of dark matter have been observed since the late 1930s. Today scientists know that luminous matter – the kind we see around us every day – accounts for just one-fifth of the matter in the universe. Dark matter makes up the rest, but scientists have yet to directly observe its building blocks.
Most prevailing theories regard dark matter as elusive, rarely interacting particles. Much of dark matter research focuses on weakly interacting massive particles, or WIMPs, which are usually theorized to be heavier than the proton. But scientists have expanded their search to account for other possibilities.
MAGIS-100 will look for ultralight dark matter candidates that weigh much less than a WIMP. An example of such a particle is the postulated axion, which would weigh less than the mass of a neutrino, a particle so light that, for decades, it was thought it would be massless.
In the case of the particles MAGIS-100 would be sensitive to, dark matter is better described as a wave, rather than a particle, causing quantities thought of as constants to oscillate with time. MAGIS-100 will search for such oscillation signals coming from the lightest dark matter candidates of this type.
When atoms are in superposition, they can become sensitive to minute alterations from ultralight dark matter particles. One such alteration occurs if such a particle interacts with an electron, which could slightly alter the electron’s mass. Ultralight dark matter particles could also affect the interaction strength of the particles that make up the atom.
“That’s why we’re so focused on how far apart we can drive an atom with MAGIS-100,” said Jason Hogan, spokesperson for MAGIS-100 and scientist at Stanford University. “That distance is directly correlated to how sensitive our experiment is.”
Testing the uniformity of gravity
The equivalence principle is one of the more well-known effects of Einstein’s theory of general relativity, which unified three-dimensional space and time. The principle states that two objects, regardless of mass, if placed in a vacuum, will fall at the same rate when placed in the same gravitational field.
Essentially, this means that gravity acts the same for all objects throughout the universe
One of the best ways to test a principle is to take it to its natural extremes, in this case the largest and smallest bodies in the known universe. Einstein’s equivalence principle has held up both for large celestial bodies and tiny atoms. MAGIS-100 will use quantum physics to push these tests to greater precision.
MAGIS-100 scientists will drop two different isotopes of the same atom, which have minuscule differences in masses, and measure the time it takes each one to fall. If Einstein’s principle holds true, the isotopes will drop at the same rate. If there is an unanticipated result, it may indicate that a new force — possibly related to dark matter — could be at work.
“Of course, we can’t count on the discovery of a new force to come out of this endeavor,” said Robert Plunkett, MAGIS-100 project manager and senior scientist at Fermilab. “But that’s the exciting thing about science: Until you check everything, you can’t be certain.”
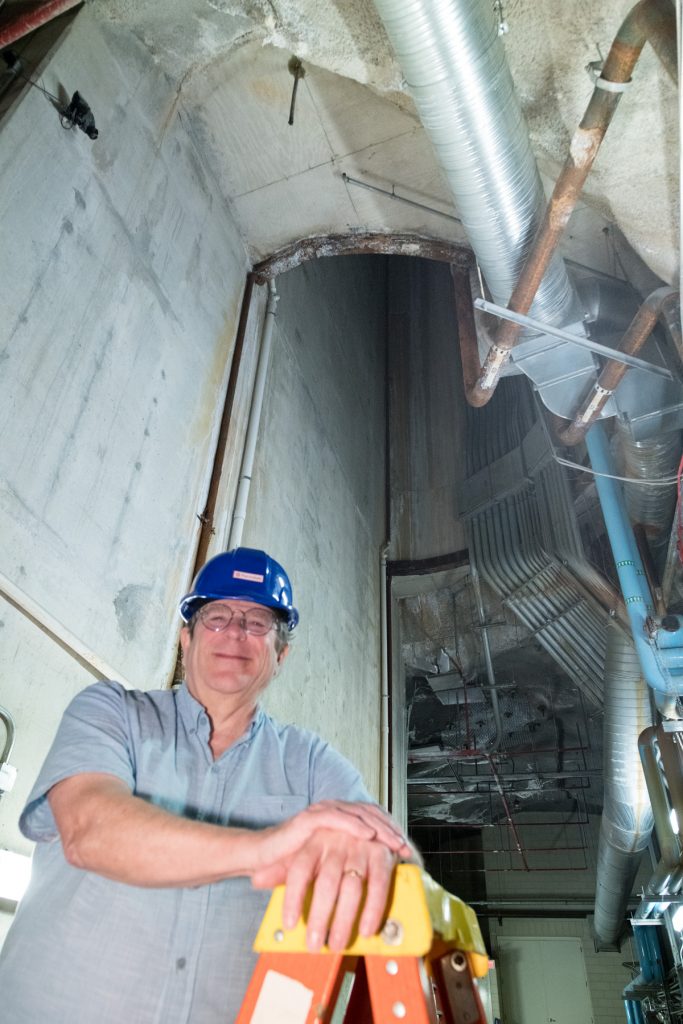
Fermilab scientist Rob Plunkett, project manager for MAGIS-100, stands in the 100-meter-deep underground experimental hall where the MAGIS-100 setup will be located. Photo: Reidar Hahn, Fermilab
New gravitational wavelengths
The first telescopes were only able to view the universe in visible light wavelengths. But starting in the 20th century, astronomers began creating telescopes to view the universe in radio, infrared, ultraviolet, X-ray and gamma-ray wavelengths. This variety of data has told scientists more about our universe than we could have ever learned with just visible light.
When the LIGO collaboration announced the discovery of gravitational waves in 2015, a nearly 100-year-old prediction made by Albert Einstein was proven right. Since then, LIGO and its sister collaboration Virgo have confirmed over 10 gravitational wave detections and have documented over 20 additional gravitational wave candidates.
But so far, scientists have been able to identify only gravitational waves within the very specific range of frequencies to which LIGO is sensitive. Scientists aim to develop instruments that will be able to test other frequencies of gravitational waves, the same way that telescopes now explore many different ranges of electromagnetic wavelengths.
The technology that MAGIS-100 uses could one day be used to discover gravitational waves with another range of frequencies. Typical gravitational wave experiments have detectors that are over 1 kilometer long. With only 100 meters to play with, MAGIS-100 scientists will not have the sensitivity to detect gravitational wave sources of the type that LIGO has seen. But their pursuit will set the groundwork for an even larger experiment that could these detect gravity wave signals at a lower frequency than LIGO, giving us more advance notice of a black hole merger before it occurs.
The next steps
In addition to Fermilab and Stanford University, scientists from four other institutions – Northern Illinois University, Northwestern University, Johns Hopkins University and the University of Liverpool – have signed on to participate in MAGIS-100.
With help from a $9.8 million grant from the Gordon and Betty Moore Foundation and funds from the DOE Office of Science’s Office of High Energy Physics through the QuantISED program, the MAGIS-100 collaboration will begin executing its plan this month, going from construction to installation to data taking.
“I personally love the beginning phases of experiments because that’s the moment when you’ve really opened the doorway to an unknown place,” Plunkett said. “And once we have the instrument, people will likely come up with even more ideas for using it.”