On Feb. 26, a crowd of engineers, technicians and analysts crowded around a computer screen as Fermilab scientist Deborah Harris pressed “stop” on the data collection for the MINERvA neutrino experiment.
“We’re all just really excited by what we’ve accomplished,” said Harris, MINERvA co-spokesperson and future professor at York University. “The detector worked wonderfully, we collected the data we need, and we did it on schedule.”
MINERvA studies how neutrinos and their antimatter twins, antineutrinos, interact with the nuclei of different atoms. Scientists use that data to help discover the best models of these interactions. Now, after nine years of operation, the data taking has come to an end, but the analysis will continue for a while. MINERvA scientists have published more than 30 scientific papers so far, with more to come. As of today, 58 students have obtained their master’s or Ph.D. degrees doing research with this experiment.
Neutrinos could hold the answer to one of the most pressing mysteries in physics: why matter was not completely annihilated by antimatter after the Big Bang. That imbalance from 13.7 billion years ago led the universe to develop into what we see today. Studying neutrinos (and antineutrinos) could uncover the mystery and help us understand why we are here at all.
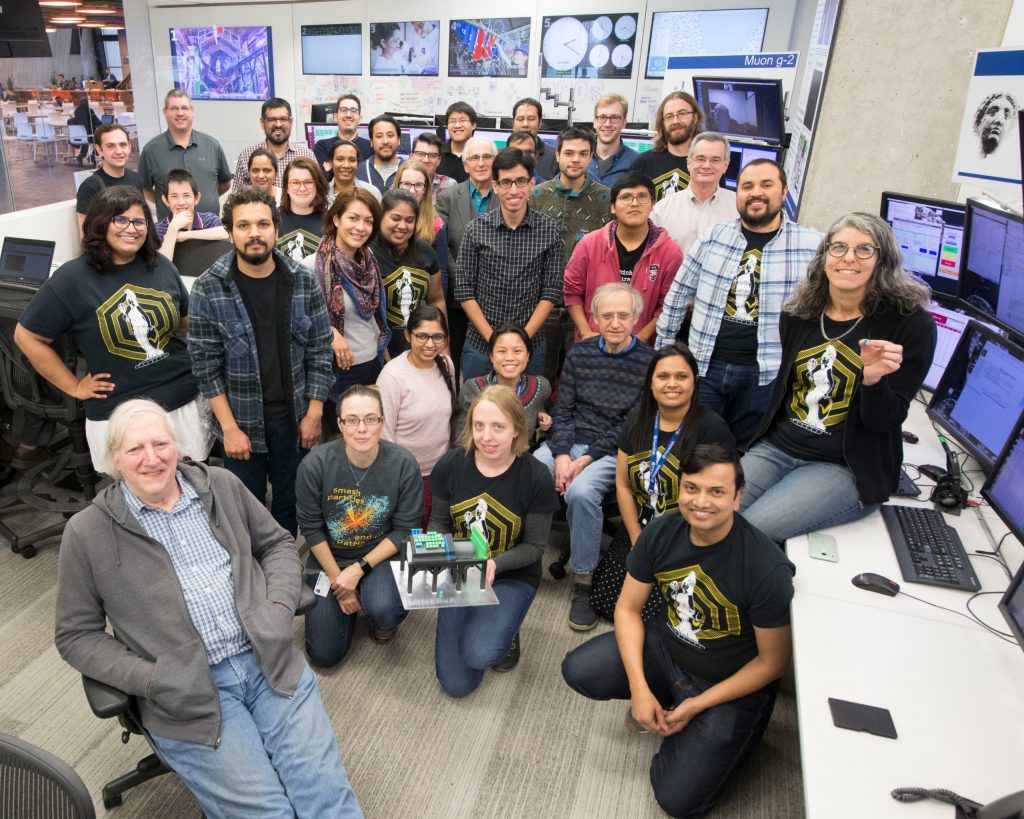
The MINERvA collaboration gathers to celebrate the end of data taking. MINERvA co-spokesperson Laura Fields, kneeling at center, holds a 3-D-printed model of the MINERvA neutrino detector. Photo: Reidar Hahn
A number of neutrino experiments investigate this mystery, including Fermilab’s NOvA experiment and the upcoming international Deep Underground Neutrino Experiment, hosted by Fermilab. To be as successful as possible, these experiments need precise models that describe what happens before and after a neutrino collides with an atom.
Every time a neutrino collides with part of an atom inside a detector, a spray of new particles flies off and travels through the rest of the detector. In order to understand the nuances of neutrinos, scientists need to know the energy of the neutrino when it first enters the detector and the energy of all the particles produced after the interaction. This task is complicated by the fact that some of the outgoing particles are invisible to the detector — and must still be accounted for.
Imagine you’re playing pool and you shoot the cue ball at another ball. You can easily predict where that second ball will go. That prediction, however, gets much more complex when your cue ball strikes a collection of balls. After the break shot, they scatter in all directions, and it’s hard to predict where each will go. The same thing is true when a neutrino interacts with a lone particle: You can easily predict where the lone ball will go. But when a neutrino interacts with an atom’s nucleus — a collection of protons and neutrons — the calculation is much more difficult because, like the pool balls, particles may go off in many different directions.
“It’s actually worse than that,” said Kevin McFarland, former MINERvA co-spokesperson and professor of physics at the University of Rochester. “All the balls in the break shot are also connected by springs.”
MINERvA provides a neutrino-nucleus interaction guidebook for neutrino researchers. The experiment measured neutrino interactions with polystyrene, carbon, iron, lead, water and helium. Without MINERvA’s findings, researchers at other experiments would have a much tougher time understanding the outcomes of these interactions and how to interpret their data.
“I really am proud of what we’ve been able to accomplish so far,” said Laura Fields, Fermilab scientist and co-spokesperson for MINERvA. “Already the world has a much greater understanding of these interactions.”
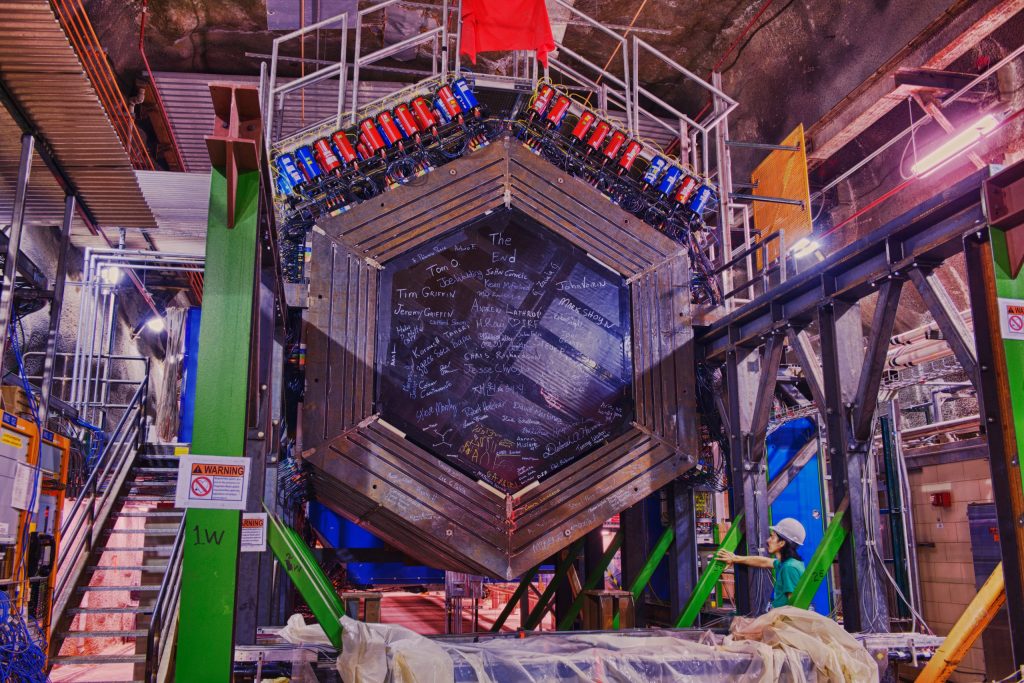
The MINERvA collaboration celebrated the completion of the neutrino detector’s construction in 2010 by signing the last plane installed. Photo: Reidar Hahn
The idea for the experiment was first submitted to Fermilab in 2002 by two separate groups — one helmed by Fermilab scientist Jorge Morfin and one by McFarland. Bringing the two ideas together enriched the experiment as a whole and ultimately gave rise to MINERvA.
From conception to construction to data collection, the MINERvA collaboration actively recruited international participation in the experiment, encouraging contributions from numerous students and collaborators from around the world – including two institutes in India and five in Latin America (Brazil, Chile, Mexico, Peru) – to bring the experiment to life. MINERvA has welcomed 41 Latin American students and four Indian students, 30 of whom have completed advanced degrees.
“It’s been a wonderful experience,” said Morfin, former co-spokesperson for MINERvA. “It’s been a successful program for the students, and for all of MINERvA.”
MINERvA started collecting data in early 2010. In 2012, scientists working on MINERvA completed their first data set, allowing them to publish their initial findings. Following that, Fermilab increased its particle beam intensity, which increased the number of neutrinos available to MINERvA. As a result, the collaboration collected 10 times the amount of data compared to the previous run. Now MINERvA has officially completed its data acquisition.
But the work isn’t done.
“We still have a lot of data to analyze,” Fields said. “Completing our run was a celebration of MINERvA’s past and, more importantly, a look towards the future of MINERvA’s science and physics as a whole.”
Visit the MINERvA website. This work is supported by the U.S. Department of Energy Office of Science.