The puzzle: understanding how nearly undetectable particles, called neutrinos, interact with normal matter. The solution? The clever MINERvA experiment, which shares its name with the Roman goddess of wisdom.
MINERvA, the Main INjector ExpeRiment for ν-A (where v is a symbol for neutrinos) is the world’s first neutrino experiment to use a high-intensity beam to study neutrino interactions with multiple nucleus sizes at the same time.
MINERvA scientists are currently looking at how low-energy neutrinos interact with ordinary matter like the protons and neutrons that make up the center of the atom. Specifically, MINERvA’s international collaboration of university and lab scientists is studying what happens when neutrinos interact with different elements from helium all the way to lead, which have different sized atomic parts.
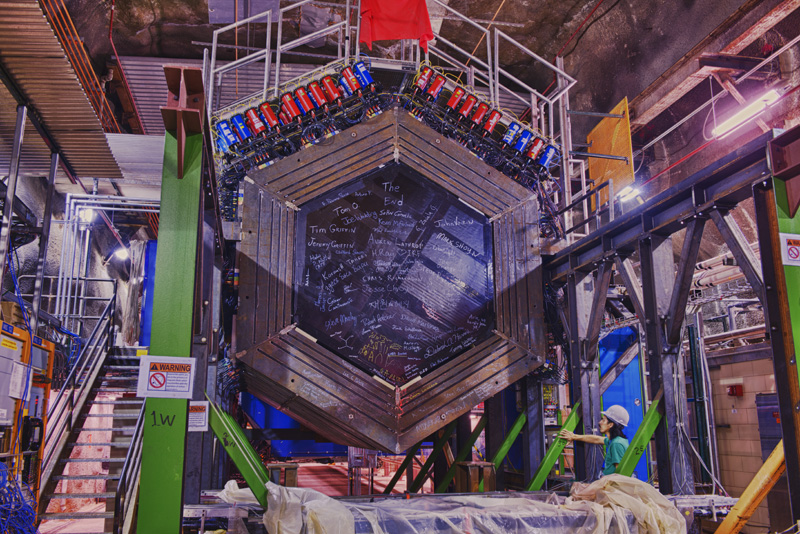
The front face of the MINERvA detector sits in its underground home near the end of construction. This front face is no longer visible because of the helium target that was installed upstream. Photo: Reidar Hahn
Imagine an atomic nucleus as racked up pool balls with little springs attached to each other and the neutrino beam as the cue ball. It’s pretty easy to see what happens if you hit the pool balls with very little energy (almost nothing happens) or a lot of energy (they all break apart). But scientists need to know what happens with neutrinos in that middle energy level.
“Some of the energy goes into breaking springs, some goes into breaking apart pool balls. Some goes into ejecting pool balls with energy,” said MINERvA co-spokesperson Kevin McFarland, a researcher at the University of Rochester. “Because it’s such a complicated system — you’re getting a big nucleus full of lots of neutrons and protons bound together with springs — it’s really hard to look at what comes out and infer precisely what the energy of the neutrino was.”
By better understanding how neutrinos interact with the matter all around us, researchers hope to improve our model of how physics — and the universe — works. The information can be used in simulations of other neutrino experiments to correct for the energy that isn’t seen in these interactions and to improve accuracy.
This information is crucial both for current neutrino experiments such as NOvA and in preparation for upcoming neutrino oscillation experiments such as the Deep Underground Neutrino Experiment, or DUNE. At the energies required for those projects, the components of the nucleus begin to break apart, producing a slew of different particles and complex data.
“We’re making measurements that haven’t been measured ever before,” said Minerba Betancourt, postdoctoral researcher for MINERvA. “For example, there’s a channel called quasielastic, in which a neutrino interacts with the detector and produces a muon and a proton. For that type of neutrino interaction, there are not any measurements of iron or lead to scintillator ratios.”
Making new neutrinos
A lot has to happen to produce MINERvA data. The experiment uses Fermilab’s Main Injector accelerator, which produces protons at energies of over 120 times their rest masses. These protons smash into a carbon target in the NuMI beamline, producing particles called pions that then transform into the desired neutrinos.
Sooner or later, a tiny fraction of these neutrinos interact with nuclei in the detector and produce daughter particles. These particles leave the nucleus, causing interactions that produce light in the scintillator detector that scientists record and analyze.
“Neutrinos are neutral, so they don’t have a charge. We can’t see them until they actually produce something,” said Daniel Ruterbories, a postdoctoral researcher for MINERvA. “All of a sudden, particles spontaneously appear.”
MINERvA has a unique ability to study neutrinos with high precision, primarily because of its detector technology. Those detector components, called scintillator bars, are small. That means physicists can measure neutrino interactions in more detail than a typical neutrino detector, which has to be huge because it has to be located hundreds of miles away from the neutrino source.
Moving forward, MINERvA will analyze higher-energy neutrinos. By taking data at about 6 GeV of energy instead of the previous 3 GeV, scientists will be able to study many more interactions in the detector.
“We’re producing a large bucket of events,” Ruterbories said. “We should be able to really focus down and try to answer the questions of how these interactions occur.”