Dark matter particles have escaped detection for decades, despite scientists’ best efforts. Now, scientists at the Department of Energy’s Fermi National Accelerator Laboratory and the University of Chicago are planning to search for these invisible particles with cutting-edge quantum sensor technology.
Researchers are currently working to prove that the technology will work as expected. Early work has been promising, and the scientists have secured a $642,000 grant from the Heising-Simons Foundation to support graduate students and over $2 million from Fermilab’s Laboratory Directed Research and Development program for equipment.
Cosmic hide-and-seek
Scientists know that dark matter exists because we can see its gravitational effects on the cosmos. The pull from dark matter affects how stars revolve in galaxies and even determines how galaxies are spread out across the sky.
In spite of its clear influence on the cosmos, scientists still don’t know what dark matter is made of.
One dark matter candidate is a theorized, fundamental particle known as the axion. Sometimes said to be named after a laundry detergent because it “cleaned up” problems in physics, the axion would be an incredibly lightweight particle that only weakly interacts with other matter. Still, with enough of them clumped together, axions could act as the dark matter that invisibly shapes our universe.
Scientists search for dark matter axions by converting them into particles of light, called photons.
“What you need is a very, very dark box,” said Aaron Chou, a Fermilab physicist who is leading the project.
According to theory, in a strong magnetic field, axions can convert into photons, which can be used as a proxy for the axions’ existence. If the magnetic-field-infused box — called a cavity — is dark enough, the scientists can observe the photons.
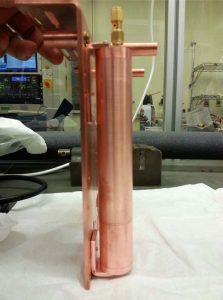
Scientists at Fermilab and the University of Chicago will look for dark matter axions converting into photons inside a sealed copper tube called a cavity. Photo: Akash Dixit
Chou’s prototype cavity is a copper tube about the size of a wine bottle. Current experiments such as the Axion Dark Matter Experiment at the University of Washington use larger cavities that are several feet in diameter.
“Traditionally, you would punch a hole in the box and stick in an antenna. That antenna is how you would read out the photons,” Chou said.
Like an FM radio, the scientists use a receiver to “listen” for axions in the cavity. By adjusting the cavity, scientists can vary the frequency — or “tune” — of the cavity and search for clear evidence of axion-to-photon conversion.
“It’s like you’re driving out in the middle of farmland, and there are hardly any radio stations at all. You’re tuning and you hear static,” Chou said. “You turn the dial a little more, you hear static, and you keep on going. Can I hear a radio station? No, just more static. You step through frequencies one at a time. That’s how the search works.”
To minimize unwanted signals, the cavity must have as few photons from other sources as possible. In other words, it has to be unimaginably dark. As it turns out, getting something to be very, very dark is very, very difficult.
A shot in the dark
To create a dark cavity, scientists eliminate all external sources of light. But even when an object is perfectly sealed, photons are still present: All matter emits photons if it has a temperature higher than absolute zero. This phenomenon is the reason that hot metal glows. Cooler objects don’t glow because the photons they emit are in the nonvisible spectrum of light.
“The colder you make something, the less likely it is to be populated by photons,” said Akash Dixit, a graduate student at the University of Chicago working on the project. “There’s exponentially less light the colder it is.”
Axion experiments are cooled all the way down to less than 1 kelvin using liquid helium. But even at these frigid temperatures, where there is virtually no noise from heat, direct measurements of particles will still have unwanted noise.
An inconvenient reality of the quantum world is that the very act of taking a measurement creates uncertainty.
“Every time you make a measurement, you’re giving the system a kick,” Chou said. “Doing that introduces an intrinsic noise into the results of the measurement.”
Normally, this isn’t a big problem. If there are millions of photons, the measurement’s uncertainty is negligible. But when scientists are looking for just one photon, things get tricky.
Chou and his team plan avoid the “kick” itself, which they can do by measuring the photons indirectly.
Q is for quantum
As a graduate student, David Schuster was a member of the first team to detect a microwave photon using a quantum sensor. Schuster, now a professor at the University of Chicago and a leading expert in quantum sensors, asked his advisor at the time if their breakthrough had any use for astronomy.
“I asked him if we could use it to detect some astronomical phenomenon,” he said. “He said, ‘Only if you can find photons that magically appear inside a sealed cavity.’”
Years later, Schuster and Chou were talking, and the two realized that they could help one another. Chou needed to observe photons appearing inside a sealed cavity, and Schuster knew exactly how to detect them.
Quantum sensors are devices that respond to incredibly small changes in electrical fields, allowing them to detect a single photon.
Because the sensors must be able to detect subtle phenomena, their construction requires a delicate touch.
Dixit uses software to design the sensors, which are then assembled using precision machines. Thin layers of material such as aluminum and niobium are added on top of one another and then engraved with lasers. Hills and valleys from these engraving and layering processes result in the sensor. The end product fits on a tiny glass slide smaller than a thumbnail.
The specially tuned quantum sensor, sitting just outside of the magnetic field that fills the dark cavity, can pick up on the presence of a photon in the cavity.
“The quantum sensor can be thought of as a guitar string that plays a particular note,” Schuster said. “Like a guitar, where pressing on a fret tunes the note by a set amount, each photon in the cavity shifts the frequency by a known amount.”
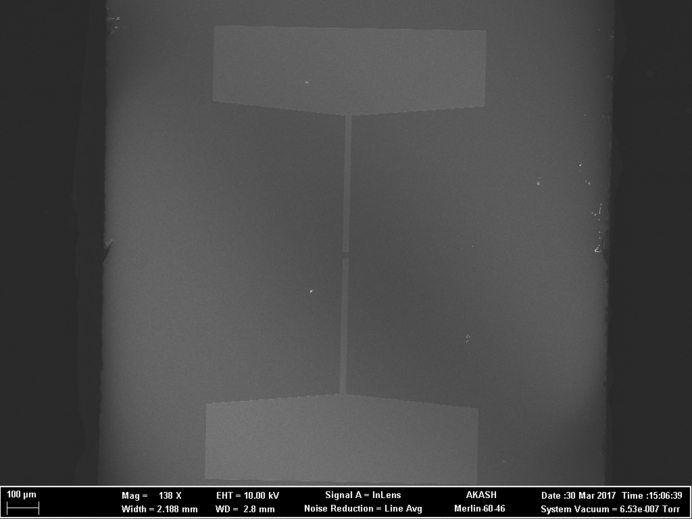
Quantum sensor GIF. See the lower left corner of the image for magnification values. Fully zoomed out (at magnification 138X), the GIF shows a quantum sensor made up of an antenna and Josephson junction, allowing scientists to “listen” for evidence of a axion converting into a photon. The large paddle-shaped regions are made of niobium and function as the antenna. Attached to the antennae are two layers of aluminum that look like funnels (magnification 1.44K and 4.39K X). This connects the Josephson junction to the surroundings. Finally, fully zoomed in (at magnification 20.44 K and 62.83 K X), the GIF shows the Josephson junction, shaped like a cross. Both pieces of the cross are made of superconducting aluminum and are separated by a thin layer of aluminum oxide (roughly 10 atoms thick). Images: Akash Dixit
The key to the sensor is the Josephson junction, formed by two overlapping strips of superconducting material only a few hundred nanometers wide — two thousandths of the width of a human hair. Pairs of electrons can hop from one side of this junction to the other.
When a photon is in the cavity, its presence changes how often — the frequency — electrons cross the Josephson junction.
Using a device with perfect pitch, the researchers will be able “hear” this change in frequency. If the change in pitch is just right, they’ll have detected a photon created by an axion.
By avoiding the “kick” of direct measurement, the scientists can indirectly and nondestructively measure the photons with lower uncertainty than typical of the quantum world.
“In simpler terms, we’re taking a device on a piece of glass and sticking it inside a cavity to ask the question, ‘Is there a photon or not?’” Chou said.
If there is a photon, then scientists could have observed an elusive axion.
Chou and Schuster plan to eventually scale the project up to have a better chance of detecting axions, using multiple cavities and more powerful magnets.
If the design works as promised, the research may graduate from project to fully funded experiment.
“Every single thing that’s part of the official lab program right now did not start out as an official thing,” Chou said. “It started out as some people with an idea, who developed that idea and had preliminary results that showed the idea wasn’t crazy.”