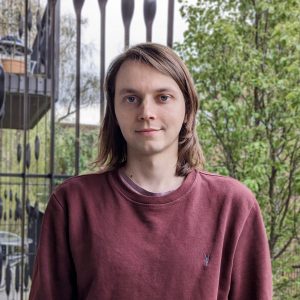
Patrick Green, who recently received his doctorate from the University of Manchester, analyzed data from a liquid argon neutrino detector, looking for theoretical particles, for his thesis research. For this work, he received this year’s URA Doctoral Thesis Award. Photo: Patrick Green
While pursuing his doctorate at the University of Manchester, Patrick Green dug into data from the past, looking for new physics with liquid argon detectors, while developing a method to improve computer modeling of results for future detectors.
Now a postdoctoral researcher at the University of Oxford, Green won this year’s Universities Research Association Doctoral Thesis Award for his work involving liquid argon time projection chambers. This annual award recognizes an outstanding doctoral thesis written on research conducted at Fermilab or in collaboration with Fermilab scientists.
“URA is excited to congratulate Patrick Green and his contributions to liquid argon time projection chamber neutrino detectors,” said URA President John Mester. “His thesis will allow higher precision neutrino measurements which will help answer some of the most pressing mysteries presented by the Standard Model.”
During his doctoral research, Green analyzed data from ArgoNeuT, a liquid argon neutrino detector at Fermilab that collected data until 2010.
“This year the committee was happy to review many excellent theses spanning the science at Fermilab,” said Chris Stoughton, chair of the URA Thesis Award Committee.
Green collaborated with theoretical physicists who were looking for insights from the ArgoNeuT data. He developed new techniques to identify the highly energetic muons of interest that would provide clues for a couple of new particles beyond the Standard Model, the current understanding of particle physics. The results of these searches set new constraints on the characteristics of these theoretical particles, Green said.
“This really close collaboration between experiment and theory is really nice, because both sides of it can be done as rigorously as you need,” he said. “You have people on the theory side that truly understand these theories and are involved in creating them, and the experiment side has a deep understanding of the detector.”
On top of the science itself, the age of the ArgoNeuT code presented its own challenges.
“I developed new techniques to select these, including developing a brand-new simulation of these models in ArgoNeuT and MINOS,” Green said. “This involved a lot of archaeology, obviously with very old detectors, digging in some very old code that no one really remembers how it works. It was challenging, but fun.”
Specifically, Green looked for signs of two theoretical particles: heavy neutral leptons, commonly called sterile neutrinos; and heavy quantum chromodynamics, or QCD, axions, a boson that would solve a mystery of the strong force.
Sterile neutrinos are a theorized new type of neutrinos beyond the known three flavors. These types of neutrinos are thought to be heavier than the very low-mass active neutrinos. They are also thought to be right-handed: other types of particles in the standard model have versions with both left-handed and right-handed spin, but all observed neutrinos so far have been left-handed.
On the lower end of the potential masses of sterile neutrinos, they could explain certain anomalies in the observations of previous neutrino experiments like the MiniBooNE experiment. Mid-mass sterile neutrinos could be a candidate for dark matter. And if sterile neutrinos have high masses, they could explain why active neutrinos are so light.
The other subject of Green’s search, QCD axions, are a solution that would explain a discrepancy between theory and observation in the strong force, known as the strong charge parity violation problem. In the weak nuclear force, the laws of physics don’t work exactly the same when particles’ charge and parity, or spatial coordinates, are flipped — in this case, CP symmetry is broken.
On the other hand, there’s no reason why charge and parity should still be conserved in interactions that use the strong nuclear force, the force that holds quarks together to make particles like protons and neutrons. For example, if CP symmetry is violated with the strong force, neutrons should have different charges on opposite sides, but this hasn’t been observed; if it’s there, it’s incredibly, and arbitrarily, small.
One solution is a new particle, the axion, which would minimize the effect of the charge asymmetry of neutrons. QCD axions could also be a candidate for dark matter.
“We don’t really have a real motivation for why CP violation in the strong force is so small compared to theory predictions,” Green said. “The axion is a very elegant model that can explain this.”
Green’s other main project during his doctoral research was developing a more computationally efficient way to model the light produced in particle interactions in liquid argon time projection chambers, or LArTPC.
“Patrick’s thesis covered both the theoretical and experimental aspects of searches for Beyond Standard Model Physics,” Stoughton said. “Specifically, his new method for simulating light production applies to all liquid argon time projection chamber experiments that are a crucial part of Fermilab’s mission.”
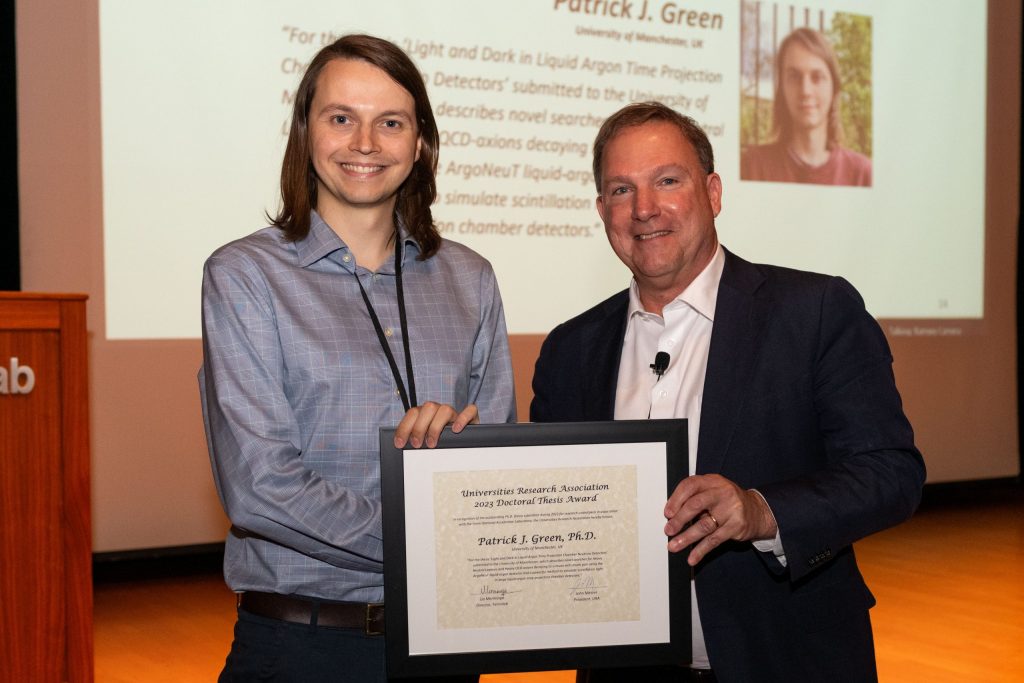
John Mester (right), URA president, presented the 2023 URA Thesis Award to Patrick Green (left) at the June 29 ceremony. Photo: Ryan Postel, Fermilab
In an LArTPC, when a neutrino hits an atom of liquid argon, light is produced in a process called scintillation. This process creates a lot of photons, and tracking each of these photons’ movements through the detector can take up a lot of computing power. A current method to help handle this computing load is a series of lookup tables that model the light in different regions of the detector. But as these detectors get bigger, such as the LArTPC neutrino detectors in the upcoming Deep Underground Neutrino Experiment, this method becomes unwieldy.
Instead, Green and his collaborators developed a new method that predicts the behavior of the light using geometric calculations. The effects of scintillation can then be treated as small corrections to that prediction. This model, which can be scaled up more easily, will be used in the Deep Underground Neutrino Experiment and the Short Baseline Near Detector.
“If you’re using a gigantic lookup table, if you double the size of your detector, you double the size of your lookup table,” Green said. “At some point, you run out of memory and computing resources to handle that large lookup table. Whereas, if you’re just making a geometric calculation each time, with some corrections, you can predict any size of detector.”
Now, Green continues to work with LArTPC detectors, but has shifted his focus to the electron flavor of neutrinos. He is also excited for the new data that will be produced by SBND and DUNE that will let researchers look for hints at something beyond the Standard Model.
“I’d like to thank my supervisor, Andrzej Szelc, for his help throughout all of this, and the many people at Fermilab and various places,” Green said. “All these things are collaborative efforts; many people have contributed to this.”
Fermi National Accelerator Laboratory is supported by the Office of Science of the U.S. Department of Energy. The Office of Science is the single largest supporter of basic research in the physical sciences in the United States and is working to address some of the most pressing challenges of our time. For more information, please visit science.energy.gov.