Long before the Deep Underground Neutrino Experiment takes its first measurements in an effort to expand our understanding of the universe, a prototype for one of the experiment’s detectors is blazing new trails in neutrino detection technology.
DUNE, currently under construction, will be a massive experiment that spans more than 800 miles. A beam of neutrinos originating at the U.S. Department of Energy’s Fermi National Accelerator Laboratory will pass through a particle detector located on the Fermilab site, then travel through the ground to a huge detector at the Sanford Underground Research Facility in South Dakota.
The near detector consists of a set of particle detection systems. One of them, known as the ND-LAr, will feature a liquid-argon time projection chamber to record particle tracks; it will be placed inside a container full of liquid argon. When a neutrino collides with one of the particles that make up argon atoms, the collision generates more particles. As each particle created in the collision travels out of the nucleus, it interacts with nearby atoms, stripping off some of their electrons, leading to the production of detectable signals in the form of light and charge. ND-LAr is optimized to see both of these types of signals. DUNE scientists chose liquid argon for one of the near detector systems so that they can make direct, one-to-one comparisons when analyzing the results from both the ND-LAr and the far detector, which also relies on liquid argon for particle detection.
The prototype for ND-LAr received its name, the 2×2 prototype, because its four modules are arranged in a square. The final version of ND-LAr will feature 35 modules, each slightly bigger than those used for the prototype. Soon, the 2×2 prototype will be installed underground in the path of Fermilab’s NuMI neutrino beam for testing.
“We’re going to put this in what is currently the world’s most intense neutrino beam,” said Juan Pedro Ochoa-Ricoux, a professor at the University of California, Irvine who is co-leading the data analysis effort for the 2×2 prototype. “We’re going to be able to test our prototype under realistic circumstances.”
Sorting a flood of neutrinos
The 2×2 prototype, and eventually ND-LAr itself, will detect the neutrino beam close to its most intense point.
When a beam of protons from an accelerator collides with a target, it creates a spray of other charged particles that quickly decay into other particles, including neutrinos. The beam of charged particles used to generate neutrinos is tightly focused, but when this neutrino beam is created, they can no longer be guided or focused, since they have no charge. As the beam travels through space, the neutrinos spread out, and the beam becomes less dense.
“It’s a little bit like a flashlight: When you aim a flashlight at a wall, if you’re close to the wall, you see a little circle, but if you get away from the wall, the circle becomes bigger and bigger and bigger,” Ochoa-Ricoux said.
Because the near detector will be close to the source of the neutrino beam, it will pick up more neutrino interactions in a smaller space than the far detector will. This powerful influx of neutrinos presents some challenges for efficiently recording the neutrino interactions in ND-LAr. While the far detector might only pick up one neutrino at the time, the near detector will see many more neutrinos interacting.
“All these interactions occur virtually at the same time,” Ochoa-Ricoux said. “We need to be able to disentangle all of these interactions.”
Fortunately, researchers at the University of Bern and DOE’s Lawrence Berkeley National Laboratory have been working on new designs and technology for a liquid-argon detector more suited to this high density of neutrinos.
The team at the University of Bern developed a novel design for liquid argon neutrino detectors. Instead of just one big volume of liquid argon, this design divides the detector into modules.
The new design not only results in a shorter distance for stripped electrons to drift toward the detecting surface, but also provides a better understanding of where the neutrino interactions are happening. Making the modules smaller shows the light produced in a neutrino interaction in one particular unit, narrowing down its location.
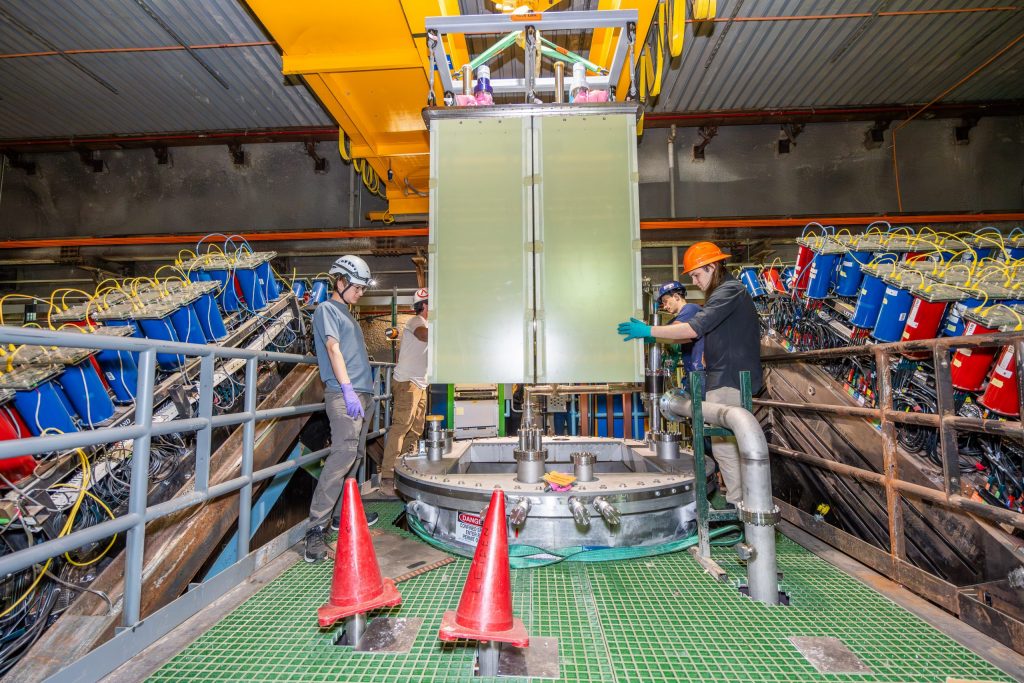
The crew moved the 2×2 prototype into place for insertion into the liquid argon cryostat, where it will collect data in the NuMI neutrino beam. Photo: Dan Svoboda, Fermilab
A modular design also means that fewer interactions take place in each module. As a result, it’s easier to pair the detection of the light and the charged particles to understand the neutrino interaction. This kind of detector can more effectively handle lots of interactions happening in a short amount of time.
These two consequences of a divided detector make it ideal for ND-LAr, as this design allows for a more accurate three-dimensional picture of where a neutrino interaction happened, said Michele Weber, a professor at the University of Bern working on the prototype detector design and leading the ND-LAr effort.
“It is great to see a concept developed at our university finding application in DUNE through a collaboration with Fermilab,” Weber said. “One challenge we had to address in order to know which signal belongs to which interaction is to improve the 3D view of each interaction.”
Getting a clearer picture
Meanwhile, at Berkeley Lab, another team has created a new type of signal readout system that can address the massive amount of data expected in the near detector.
Traditionally, liquid-argon time projection chambers, or LArTPC, have used a series of layered wires across the side of the detector to catch the signal from stripped electrons that are released in an interaction between a neutrino and the argon. Combining the signals collected by the layers of wires, which provide a series of two-dimensional projections, supplies enough information to reconstruct a three-dimensional picture of the interaction.
However, when there are a lot of neutrino-argon interactions in the detector — a phenomenon called neutrino pileup — this readout system doesn’t provide as clear of a picture, said Brooke Russell, a Chamberlain fellow at Berkeley Lab working on the 2×2 prototype.
Instead, the readout system developed at Berkeley Lab uses a fully pixelated readout, which means each physical channel in the detector corresponds to one digital readout channel. Using this array of pixels directly shows the three-dimensional location of the interaction and can resolve all of the many neutrino interactions happening nearly simultaneously.
“This has major implications for the types of signals that we construct and the intensity of activity that we can be tolerant to,” Russell said. “With the DUNE near detector, for the first time, we’re in a regime where we have neutrino pileup. Such a readout is absolutely required in order to reconstruct the neutrino events.”
Putting 2×2 to the test
The modules for the prototype were built and tested at the University of Bern, then shipped to Fermilab and tested again before their installation. Preparations are underway for the prototype’s installation by the end of the year in order to test the neutrino detection when the NuMI beam turns back on this winter.
The experiment’s installation team will place the prototype detector in a cryogenically cooled container and sandwich it between two repurposed detector pieces from the retired MINERvA neutrino experiment at Fermilab. MINERvA measured neutrino interactions from 2010 until 2019.
As the ND-LAr prototype detector isn’t very big, it cannot measure the full path of some of the particles created when neutrinos interact with argon. Notable examples are muons, which typically travel for long distances before stopping. That’s where the old MINERvA detector components come into play. Using those components to track muons exiting the prototype detector, scientists can distinguish muons from charged pions, another type of subatomic particle.
Placing the prototype between the MINERvA segments also helps identify muons that are passing through but didn’t originate in the detector, distinguishing them from the muons coming from inside the detector as a product of neutrino interactions.
“We can use the MINERvA planes to help us track neutrinos that interacted in the rock upstream of the detector and made muons that went into the detector,” said Jen Raaf, director of the Neutrino Division at Fermilab who coordinates the 2×2 prototype project. “We’ll be able to connect the tracks to identify those [that didn’t originate in the detector] and get rid of them, because that’s not what we’re interested in.”
The MINERvA planes also allow the scientists to track particles created in neutrino interactions in the LArTPC, but which exit the argon volume before stopping. “MINERvA will allow us to track these exiting particles and measure their energy,” Raaf said, “so that we can get an accurate estimate of the neutrino’s energy when it interacted in the LArTPC.”
When the 2×2 prototype is tested in the neutrino beam, not only will it ensure that the prototype is working properly, but researchers can also perform neutrino physics experiments, Ochoa-Ricoux said.
Even though the full-blown DUNE experiment won’t start operating for several more years,” he said, “we’re already going to be producing some important physics results with this prototype.”
Some of these pre-DUNE experiments in the 2×2 prototype include studying the reactions between neutrinos and the argon, and measuring cross sections, or the likelihood of particle interactions.
Between the modular design and the pixel readout, ND-LAr will be unique among liquid argon neutrino detectors. This means that building and testing a prototype is crucial to ensuring that the innovative design works as expected. As a new piece of technology is built, scientists must test each step of the construction to demonstrate its capabilities, Weber said.
“ND-LAr has an atypical design,” Russell said. “We want to validate that some of the design principles that we think will work, will actually work.”
It’s also important that a prototype is built big enough to ensure that the final piece of equipment is possible to construct and install, Raaf said.
“Doing something on a smaller scale, but big enough that you would be able to identify difficulties in construction and assembly, is a really important step of all particle physics experiments,” she said. “You want something that’s big enough to experience the various things you have to do, like use a crane to pick it up and be able to move it in certain ways.”
The DUNE collaboration is organized into consortia that focus on different aspects of the project. The development of the 2×2 prototype is part of the ND-LAr Consortium, of which the University of Bern and Berkeley Lab are only two among dozens of institutions.
“All of those people are participating in this prototype at some level, to make sure that what they’ve envisioned for the full-sized thing actually works on a smaller scale and that we don’t need to tweak anything,” Raaf said. “Maybe we will, which is fine — that’s why we do prototypes. We meet weekly and discuss, how’s it going? What do we need to do next? What went well? What can we improve?”
For a task this big, collaboration between multiple institutions is necessary, said Weber, who serves as the lead of the ND-LAr Consortium. Between Fermilab’s neutrino beam, the University of Bern’s modular detector concept, Berkeley Lab’s readout technology, and the data processing and analysis taking place at many institutions, each collaborator in the ND-LAr Consortium brings its unique capabilities to bear on this project.
“These efforts are too big for one institution alone,” Weber said. “You talk to various people, and you share the load. It’s a challenge to work with a lot of people, but it’s the only way, and it’s nice to see different ideas come together successfully.”
Fermi National Accelerator Laboratory is supported by the Office of Science of the U.S. Department of Energy. The Office of Science is the single largest supporter of basic research in the physical sciences in the United States and is working to address some of the most pressing challenges of our time. For more information, please visit science.energy.gov.