Para una versión en español, haga clic aquí. Para a versão em português, clique aqui.
Scientists solve neutrino mysteries by watching them interact with detectors — specifically, with the atomic nuclei in the detector material. Most of the time, a neutrino does not even shake hands with a nucleus. But when it does, the lightweight, neutral particle can transform into a charged particle and knock things out of the nucleus as it escapes — leaving a crime scene behind. It is the job of scientists at Fermilab’s MINERvA experiment to reconstruct the crime scene and figure out what has happened during the interaction.
The impact
Neutrinos are lightweight particles that rarely interact with matter. Their reluctance to interact makes them difficult to study, but they’re also the very particles that could answer longstanding questions about the creation of the cosmos, so they’re worth the pursuit. And it’s a tough one, since the neutrino can’t be studied directly. Rather, scientists must study the traces it leaves behind. The more information they can gather about the meaning of those traces, the better their neutrino measurements — not just at MINERvA, but at other neutrino experiments as well.
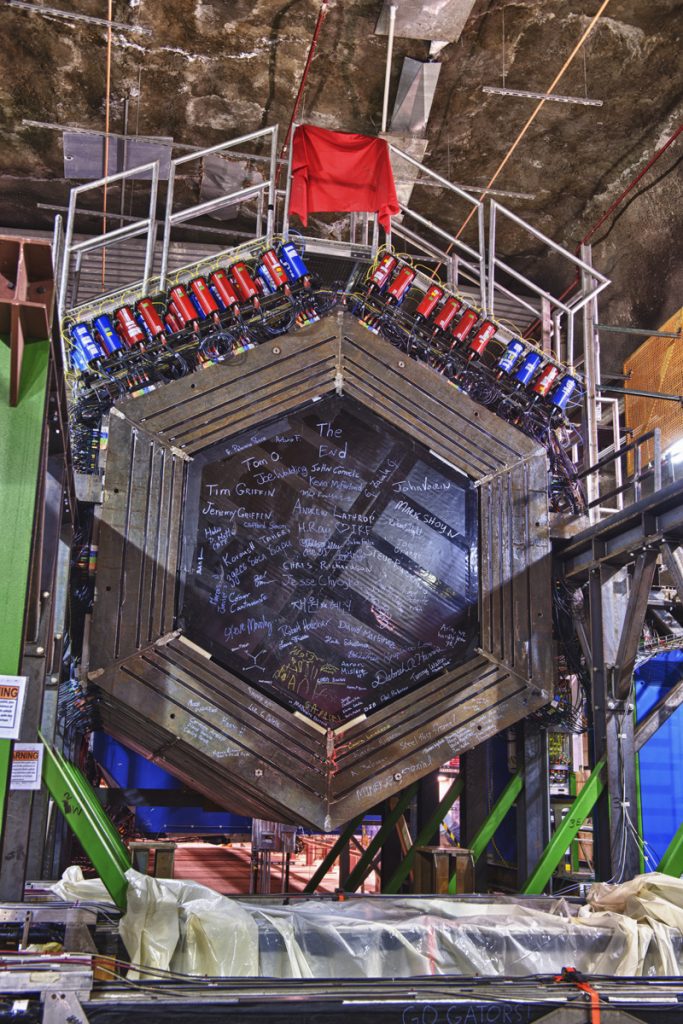
Neutrinos entering the MINERvA detector interact with the detector’s atoms, generating new particles before fleeing the scene. The MINERvA experiment recently used a new investigative technique to better trace those fleeing neutrinos that kicked everything off. Photo: Reidar Hahn
Summary
Neutrinos are lightweight, neutral particles, and they usually sail through matter without bumping into it. But once in a while, it does shake hands with a nucleus, and sometimes the handshake takes a destructive turn: A charged lepton (an electron or muon, sometimes called a “heavy electron”) is produced, while the constituents of the nucleus are knocked out. The traces of the charged lepton and the knock-out are collected by a particle detector.
MINERvA scientists study the resultant particles’ traces to reconstruct the interaction between the neutrinos and the nuclei. So far, this has not been an easy task: Nuclear effects have obscured much of the evidence of the intruding neutrinos, leaving researchers with complex and seemingly irrelevant information. Not all neutrinos misbehave but, unfortunately, the neutrinos we care about – those with energy comparable to the mass of the constituents of the nuclei and could possibly tell us about the creation of the cosmos – all have this modus operandi.
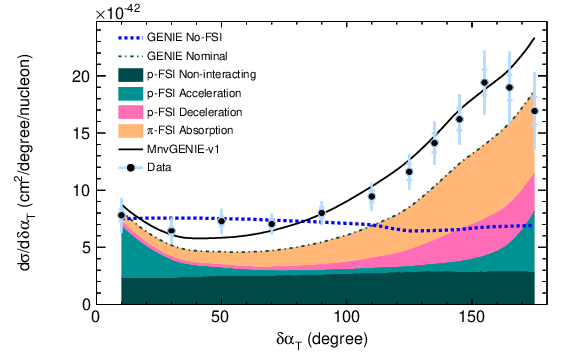
The transverse boosting angle δαT represents the direction of the net transverse motion of the charged lepton and the knock-out.
To reconstruct the resulting crime scene, scientists need a complete understanding of how the nuclear effects work.
Both the charged lepton and the knock-out retain partial fingerprints from the original neutrino, and those partial fingerprints lie ambiguously on top of the nuclear effect background.
Researchers have found that the fingerprints can be lifted via a novel neutrino CSI technique known as “final-state correlations.” Just as the sun’s corona is visible only during a solar eclipse, the fine details of the nuclear effects become clear only when other effects are removed.
To get a sense of the “final-state correlations” technique, let’s take a step back and look at the events leading to the crime scene: A neutrino bumps into a nucleus. The interaction produces other particles. Those new particles — charged lepton and knock-out — fly off in opposite directions, leaving traces of themselves in the detector.
In the absence of nuclear effects, the charged lepton and the knock-out would fly off in separate, roughly back-to-back paths, away from the incoming neutrino path. Picture a neutrino entering through, say, the south entrance of some tiny, subatomic building. It bumps into a nucleus. The resulting charged lepton flees through an east exit, and the knock-out particle flees through some west exit.
With no nuclear effects, the charged lepton heads east with as much determination as the knock-out particle heads west. That is, the charge lepton’s east-pointing momentum matches the knock-out particle’s west-pointing momentum.
But in reality, there are nuclear effects, and that means that the charged lepton’s eastward motion does not match the knock-out particle’s westward motion. These subtle momentum differences are clues; they reflect everything that happens inside the nucleus, like a shadow of the crime scene cast by the flashlight carried by the neutrino. Thus, neutrinos cast no shadows – only nuclear effects do.
The final-state correlations technique matches the nuclear effects with the postinteraction particles’ departures from the paths of equal east-west momenta.
In a recent MINERvA neutrino investigation, researchers used the new technique. They laid out a detailed reconstruction of the nuclear effects. The underlying phenomena – such as the initial state of the nucleus, additional knock-out mechanism, and final-state interactions between the knock-out and the rest of the nucleus – are now separated. New insights on the workings of nuclear effects have been reported in Phys. Rev. Lett. 121, 022504. Those interested are much encouraged to review MINERvA’s findings.
Xianguo Lu is a physicist at the University of Oxford.