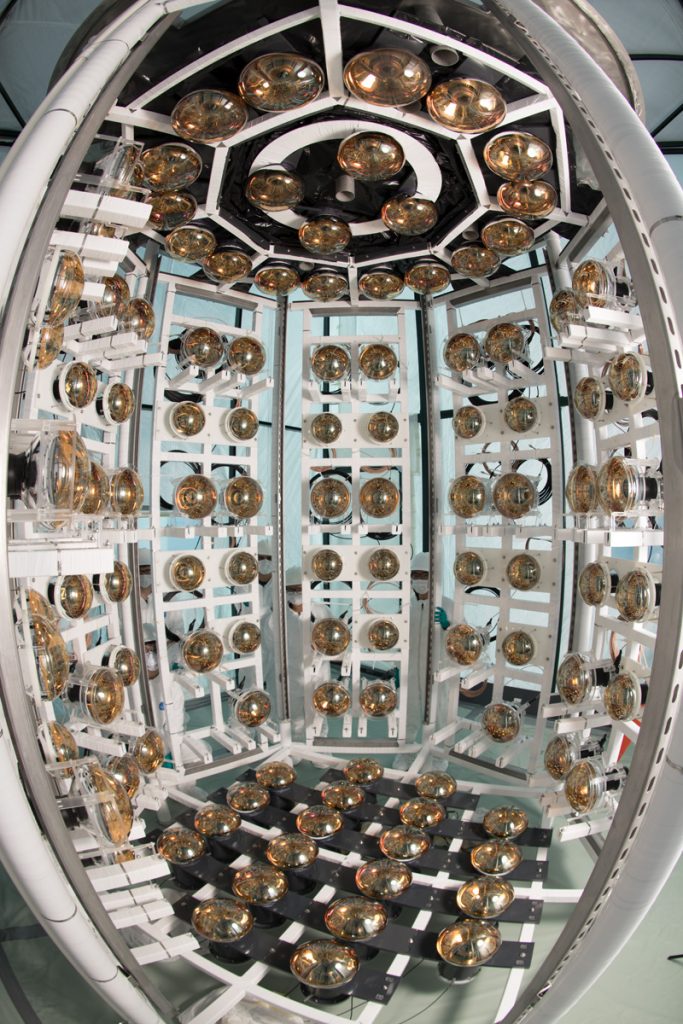
Photomultiplier tubes dot the 26-ton water tank of ANNIE, the Accelerator Neutrino Neutron Interaction Experiment. Photo: Reidar Hahn, Fermilab
The inside of the ANNIE detector looks like a series of carefully placed Jell-O domes, or perhaps a jeweled Fabergé egg. Its walls are dotted by 137 sensors for detecting packets of light and embrace 26 tons of gadolinium-doped water.
Starting in December, beams of particles called neutrinos will hurtle toward the ANNIE detector at Fermilab, strike atoms inside it and knock off neutrons, shedding light on how neutrinos interact with atoms in a water target and how they scatter.
The physics
Neutrinos are among the most abundant particles in the universe. We know that they have mass and that they oscillate among other neutrino types. But what is this mass? How do neutrinos oscillate? And how do neutrinos interact with nuclei, the core of atoms?
A crucial step in answering these questions is determining the energy of the neutrino that caused a collision. But to do so, physicists only can look at the particles that emerge after the collision took place. It’s like determining the speed of a bowling ball by just looking at the pins it hit.
“Any byproduct of neutrino interactions – neutrons, protons, muons – is important because physicists want to reconstruct the neutrino’s energy,” said Emrah Tiras, ANNIE Phase II detector upgrade manager at Fermilab and postdoctoral researcher at Iowa State University.
In water, neutrinos are typically observed indirectly via the blue Cherenkov light emitted by electrically charged particles that are produced in a neutrino’s interaction with an atomic nucleus. By identifying the locations and times each light photon is detected, physicists can reconstruct the subatomic scene, determining when and where the corresponding charged particle was emitted and in turn learn about the neutrino and interaction that produced the charged particle in the first place.
The problem is that neutrons are not charged particles. They are electrically neutral, meaning they don’t produce Cherenkov light. Because of this, they are hard to detect and easy to go unnoticed.
“Events with charged particles, like protons and muons, are easier to reconstruct, but generally most events include a mixture of charged and neutral particles. Without knowledge of the neutron component in any given event, you can miscalculate the neutrino energy,” Tiras said.
But ANNIE has a solution. The experiment will detect and discriminate between signals produced through two neutrino-nucleus interaction pathways. The first is the transformation of a muon neutrino into a muon. The second is the production of a neutron, which interacts in the water target and produces detectable gamma rays.
Physicists developed and deployed two innovative technologies on ANNIE to address these major uncertainties, count the number of neutrons and determine the probability of neutrino-nucleus interactions.
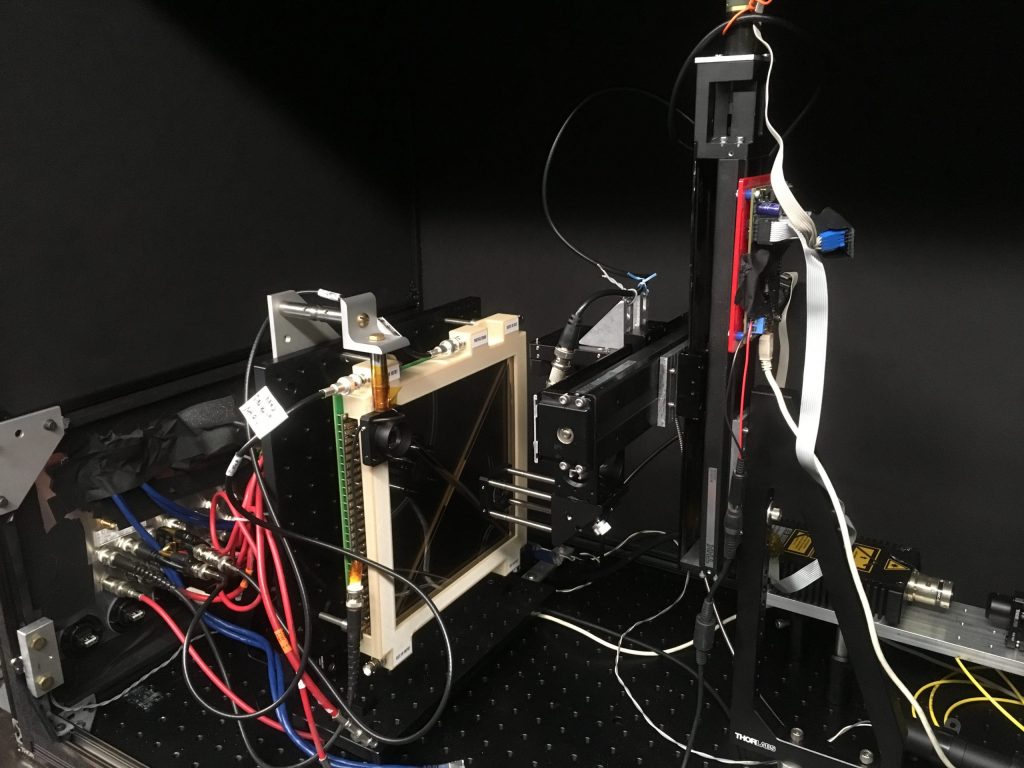
This test setup is used to characterize large-area picosecond photodetectors for the ANNIE neutrino experiment. Photo: Emrah Tiras
Two technologies
ANNIE will be the first neutrino experiment to employ a new generation of imaging photodetectors.
Large-area picosecond photodetectors, or LAPPDs, detect light, retrace its origins and determine the time the light was emitted. Whereas the more common technology, photomultiplier tubes, might detect “blobs,” or packets, of charge coming from separate photons in a neutrino-nucleus interaction, LAPPDs detect single photon arrivals separated by approximately 60 trillionths of a second. This corresponds to the time it takes light to travel a little over 1.5 centimeters, improving performance over photomultiplier tubes by a factor of 100. ANNIE will detect signals with five LAPPDs and 132 photomultiplier tubes.
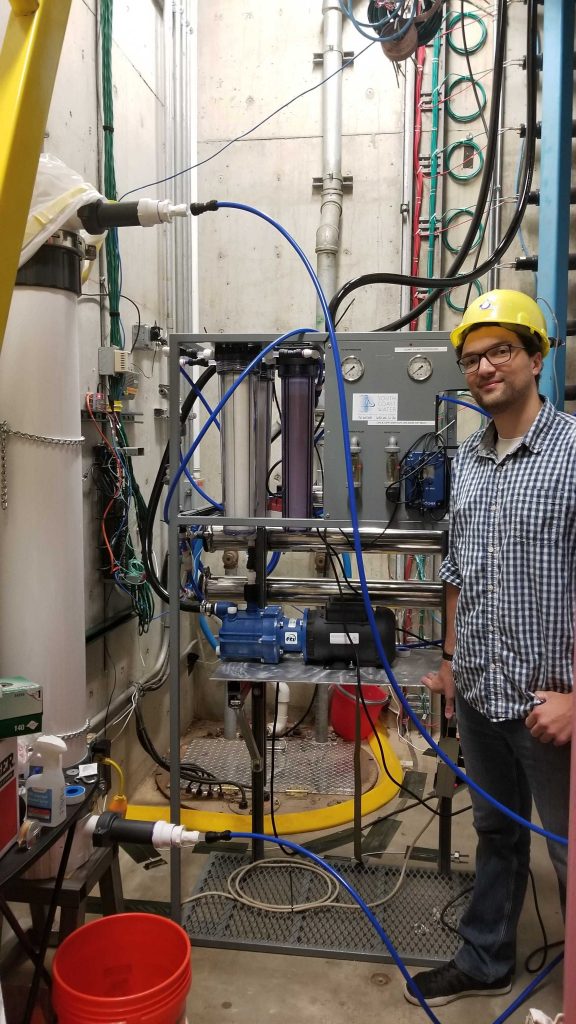
Vincent Fischer, postdoctoral researcher at University of California, Davis, stands next to ANNIE’s water filtration system in the experimental hall at Fermilab. Photo: Emrah Tiras
Physicists still needed to tag individual neutrons, and gadolinium-enhanced water is the solution.
Gadolinium, a relatively inexpensive metal used in magnetic resonance imaging and superconductors, forms salts that are dissolvable in water. Adding gadolinium will increase the likelihood of neutron interactions by approximately 150,000 times over pure water alone.
“Once a neutron is captured by gadolinium, a shower of gamma rays is emitted that can then be detected by photomultiplier tubes and LAPPDs,” said Vincent Fischer, postdoctoral researcher at the University of California, Davis, who works on ANNIE. “Adding gadolinium does make cleaning the water more challenging, though.”
Signals in water-based Cherenkov detectors can be absorbed or obstructed by dust, bacteria and other materials, requiring that physicists regularly clean, or purify, the water. But the cleaning systems used in large experiments tag gadolinium, essential to ANNIE, as a contaminant. Fischer and his colleagues at the University of California, Davis, developed a new resin and a new system to circulate and purify the water without filtering out the gadolinium.
Another challenge for Fischer was scheduling when and how much gadolinium should be added to the water.
“If you add gadolinium to the water too soon, rust can form. It’s like ships, which rust faster in seawater than freshwater,” Fischer said.
ANNIE currently holds around 1% of its ultimate gadolinium load, which corresponds to a 10% efficiency in capturing neutrons. The collaboration aims to hit 100% of the planned gadolinium load, or 90% neutron capture efficiency, in the next few weeks.
Today and tomorrow
Together, photomultiplier tubes, LAPPDs and gadolinium-enhanced water will allow ANNIE physicists to accurately identify and count neutrons from neutrino-nucleus interactions. In addition, ANNIE will have a fully operational muon range detector, which analyzes signals originating from muons arising from neutrino-nucleus interactions and escaping the main detector.
The collaboration plans to start taking physics data – detecting neutrons originating from neutrino interactions and measuring the probabilities of neutrino interactions in water – in early December.
“This effort is a culmination of almost eight months of work with, on any given week, four or five collaborators working on detector construction, installation, testing and calibration systems,” said Ashley Back, ANNIE postdoctoral researcher at Iowa State University. “We’re excited to share this achievement with the Fermilab community and the public.”
After the current phase of upgrades and data collection are complete, physicists want to continue using ANNIE as a testbed for new technologies, and the results will be used by larger neutrino experiments to benchmark and constrain their own measurements.
An international collaboration of more than 30 individuals, ANNIE is certainly ready to close the gap on unknowns in neutrino-nucleus interactions, yielding important information for neutrino scientists around the world.
This work is supported by the Department of Energy Office of Science.