Para una versión en español, haga clic aquí. Para a versão em português, clique aqui. Pour une version en français, cliquez ici.
Neutrinos are the most abundant elementary particles in the universe that weigh something. They have no electric charge, and they pass through almost everything since they interact only weakly with matter. There are three types of neutrinos, and they change from one type into another other as they travel, a behavior called oscillation. Physicists have been engaged for years in measuring how much and how often neutrinos oscillate between their various types.
Our understanding of the process crucially depends on the measurement of the neutrino energy. We cannot measure the energy of the neutrinos themselves; we can measure only the energies of the particles that are produced by the interaction of a neutrino with the detector, typically made of material of heavy nuclei, which enables lots of interactions and so opportunities for observing the neutrino’s behavior. The nuclear environment is a complicated one, so making measurements is a difficult task.
We need a detailed understanding of the neutrino’s interaction inside the nucleus to precisely measure its energy. In the energy range of many oscillation experiments, the dominant interaction process is one in which a neutrino completely scatters off a neutron inside the nucleus and produces a muon and proton. The produced proton can interact further with other particles inside the nucleus. Thus in the presence of other protons and neutrons, the distributions of the final-state particles may differ from the primary interaction.
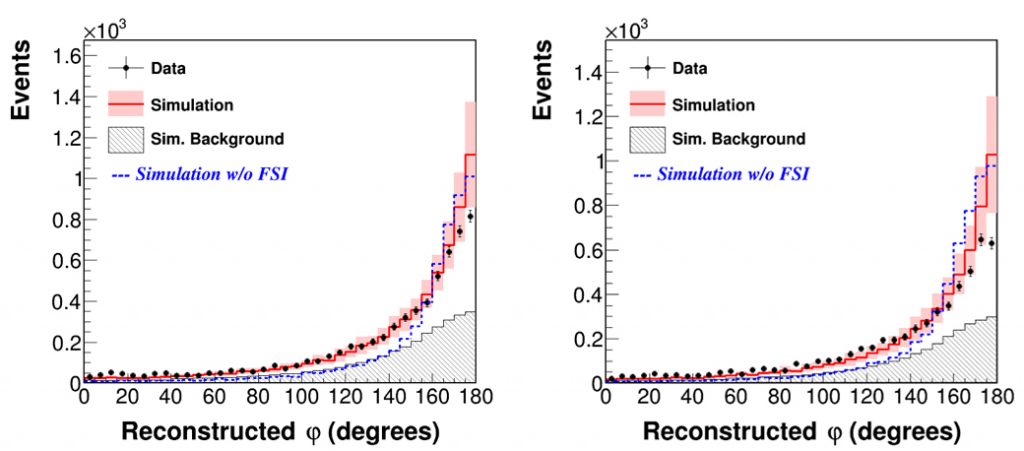
These plots show the angle between the plane of the neutrino-proton interaction and that of the neutrino-muon interaction for iron (left) and left (right).
One way to see how the nucleus changes the picture is to look at the angle between two planes that define the interaction: If you think of the plane that includes the neutrino direction and the proton direction, and also the plane that includes the neutrino direction and the muon direction, those two planes should be “back to back,” or different by 180 degrees. MINERvA can measure the angle between those two planes precisely. It turns out that these events are not all at 180 degrees apart — not even close.
If you are a teacher, you have to deal with this kind of situation every day. Suppose you supervise a large group of kids, and you know the individual behavior of each kid. However, when they are in a large group, each kid’s behavior is affected by others, so you adjust your prediction. The behavior of a kid in a group will be different from her behavior when she is by herself.
Think of the proton and neutrons as the kids, which are inside a bound nucleus. Your estimation of how much energy the kids have will be different once you take into account the fact that they are losing lots of energy interacting with each other, compared to when they were just sitting in separate rooms by themselves.
MINERvA recently made a new measurement of this process by including all the events with a muon plus at least one proton and no lighter particles. The probability of a neutrino interaction is measured as a function of momentum transferred to the nucleus (called “Q2”), which is calculated using a measurement of the proton’s energy.
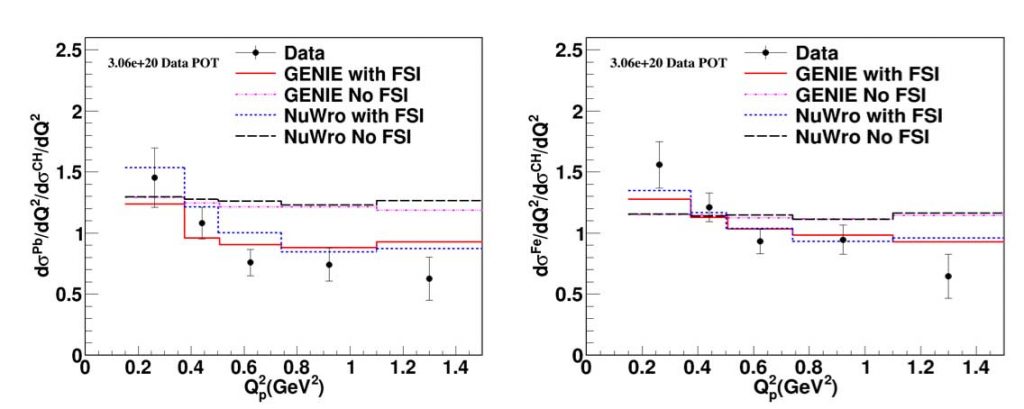
These graphs show the ratio between the cross section on lead (left) and iron (right) to the cross section on hydrocarbon (plastic).
MINERvA carried out the measurement simultaneously on carbon, iron and lead. A previous MINERvA study measured this process on plastic (hydrocarbon) alone.
If all the kids in the nucleus behaved like individuals, then the ratio of cross sections would be close to a constant that’s related to ratio of the fraction of neutrons in each target nucleus. But these ratios are not at a constant value, as you can see in the plots.
This measurement also shows that the dependence on the target element (lead or iron) is not well-described in the current nuclear models and that the theorists have some homework to do before the predictions for experiments measuring oscillations will be precise. This is the first time a direct measurement of this has ever been made. This result was submitted for publication last week.
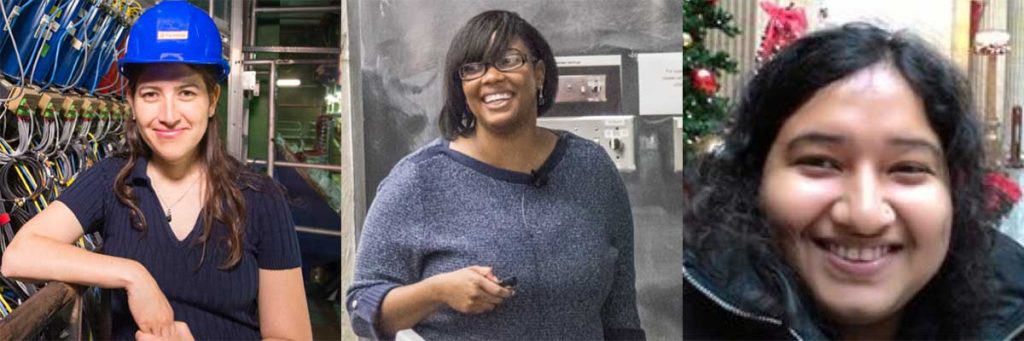
These physicists lead this analysis: Minerba Betancourt (left) and Tammy Walton (middle) of Fermilab, and Anushree Ghosh (right) of Federico Santa María Technical University.
Anushree Ghosh is a postdoctoral researcher at Federico Santa María Technical University.