An experiment at the Department of Energy’s Fermilab has made a significant advance in the detection of neutrinos that hide themselves at lower energies.
The ArgoNeuT experiment recently demonstrated for the first time that a particular class of particle detector — those that use liquid argon — can identify signals in an energy range that particle physicists call the “MeV range.” It’s the first substantive step in confirming that researchers will be able to detect a wide energy range of neutrinos — even those at the harder-to-catch, lower energies — with the international Deep Underground Neutrino Experiment, or DUNE, hosted by Fermilab. DUNE is scheduled to start up in the mid-2020s.
Neutrinos are lightweight, elusive and subtle particles that travel close to the speed of light and hold clues about the universe’s evolution. They are produced in radioactive decays and other nuclear reactions, and the lower their energy, the harder they are to detect.
In general, when a neutrino strikes an argon nucleus, the interaction generates other particles that then leave detectable trails in the argon sea. These particles vary in energy.
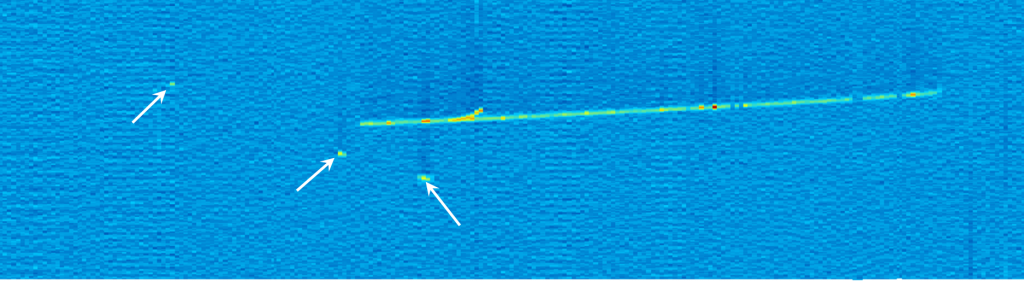
This is a visual display of an ArgoNeuT event showing a long trail left behind by a high energy particle traveling through the liquid argon accompanied by small blips, indicated by the arrows, caused by low energy particles.
Scientists are fairly adept at teasing out higher-energy particles — those with more than 100 MeV (or megaelectronvolts) — from their liquid-argon detector data. These particles zip through the argon, leaving behind what look like long trails in visual displays of the data.
Sifting out particles in the lower, single-digit-MeV range is tougher, like trying to extract the better hidden needles in the proverbial haystack. That’s because lower-energy particles don’t leave as much of a trace in the liquid argon. They don’t so much zip as blip.
Indeed, after simulating neutrino interactions with liquid argon, ArgoNeuT scientists predicted that MeV-energy particles would be produced and would be visible as tiny blips in the visual data. Where higher-energy particles show as streaks in the argon, the MeV particles’ telltale signature would be small dots.
And this was the challenge ArgoNeuT researchers faced: How do you locate the tiny blips and dots in the data? And how do you check that they signify actual particle interactions and are not merely noise? The typical techniques, the methods for identifying long tracks in liquid argon, wouldn’t apply here. Researchers would have to come up with something different.
And so they did: ArgoNeuT developed a method to identify and reveal blip-like signals from MeV particles. They started by comparing two different categories: blips accompanied by known neutrino events and blips unaccompanied by neutrino events. Finally, they developed a new low-energy-specific reconstruction technique to analyze ArgoNeuT’s actual experimental data to look for them.
And they found them. They observed the blip signals, which matched the simulated results. Not only that, but the signals came through loud and clear: ArgoNeuT identified MeV signals as a 15 sigma excess, far higher than the standard for claiming an observation in particle physics, which is 5 sigma (which means that there’s a 1 in 3.5 million chance that the signal is a fluke).
ArgoNeuT’s result demonstrates a capacity of crucial importance for measuring MeV neutrino events in liquid argon.
Intriguingly, neutrinos born inside a supernova also fall into MeV range. ArgoNeuT’s result gives DUNE scientists a leg up in one of its research goals: to improve our understanding of supernovae by studying the torrent of neutrinos that escape from inside the exploding star as it collapses.
The enormous DUNE particle detector, to be located underground at Sanford Lab in South Dakota, will be filled with 70,000 tons of liquid argon. When neutrinos from a supernova traverse the massive volume of argon below Earth’s surface, some will bump into the argon atoms, producing signals collected by the DUNE detector. Scientists will use the data amassed by DUNE to measure supernova neutrino properties and fill in the picture of the star that produced them, and even potentially witness the birth of a black hole.
Particle detectors picked up a handful of neutrino signals from a supernova in 1987, but none of them were liquid-argon detectors. (Other neutrino experiments use, for example, water, oil, carbon or plastic as their detection material of choice.) DUNE scientists need to understand what the lower-energy signals from a supernova would look like in argon.
The ArgoNeuT collaboration is the first experiment to help answer that question, providing a kind of first chapter in the guidebook on what to look for when a supernova neutrino meets argon. Its achievement could bring us a little closer to learning what these messengers from outer space will have to tell us.
This work is supported by the DOE Office of Science.