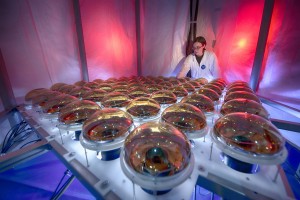
Carrie McGivern prepares photomultiplier tubes during the ANNIE detector assembly. Photo: Reidar Hahn
If you’ve passed by the old SciBooNE hall at Fermilab in the last couple of months, you might have heard a bit of commotion. A little experiment with big ambitions just finished moving in this week after a year’s worth of planning and research. The Accelerator Neutrino-Neutron Interaction Experiment, called ANNIE, recently settled down and began taking phase one data on April 15.
“We want to better understand the nature of neutrino interactions by looking at their effects on an atom’s nucleus,” said Matthew Wetstein, a co-spokesperson for the ANNIE project and a Fermilab visiting scientist from Iowa State University. ANNIE will be the first realization of a new kind of detector in a large neutrino experiment. The technology will help look for particle interactions that are hard to distinguish in other detectors, he said.
The ANNIE team aims to study phenomena and techniques relevant to neutrino energy and proton decay measurements through the use of a water Cherenkov detector loaded with a chemical element called gadolinium and surrounded by never before used photosensors called large-area picosecond photodetectors (LAPPDs).
Light travels slower in a medium such as glass, water or other transparent materials than in air or in a vacuum. Sometimes, light travels slow enough in these materials that particles can overtake it. That’s why Cherenkov detectors are common in neutrino experiments. When neutrinos hit atoms in such media, the resulting free-flying electrically charged particles emit their own light, known as Cherenkov radiation, and these detectors record this light, allowing scientists to identify the type of particle and calculate its energy. In ANNIE’s case, neutrinos streaming down the Fermilab Booster Neutrino Beam will strike water molecules in the detector and knock off neutrons. Neutrons are electrically neutral and do not emit Cherenkov radiation, so they need to be detected some other way. After the initial neutrino-nucleus collisions, the gadolinium salts in the water effectively capture the neutrons and subsequently emit photons, which can be detected by photosensors.
“Neutrons have always been a challenging particle to detect,” Wetstein said. “We hope ANNIE can determine how many neutrons are produced when neutrinos interact.”
Observing this neutron release is useful because neutrons carry some energy with them that was transferred from the neutrino collision. Physicists believe higher-energy neutrino-nucleus interactions produce a larger number of knocked-off neutrons. To test this, ANNIE physicists will use accelerator-born neutrinos that have an energy level similar to atmospheric neutrinos, which have some of the highest-energy yields. The ANNIE team aims to understand how tagging free-flying neutrons can help them differentiate between a possible proton-decay signal and background noise from the neutrinos.
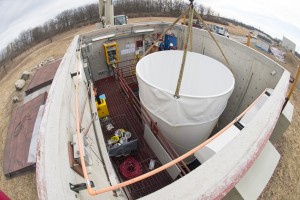
The ANNIE detector was lowered into the SciBooNE Building at Fermilab on February 29. Photo: Reidar Hahn
One of the best ways for the team to determine the effects of neutron tagging is to use the LAPPDs, a photosensor technology that researchers had been developing for nearly five years prior to the 2015 proposal for ANNIE. These sensors are currently in the commercialization phase.
LAPPDs are based on a technology called microchannel plates, which are tiny arrays with densely packed, tinier tubes that detect light. Conventional photodetectors, for physics research and commercial use, have single-pixel resolutions. In large neutrino experiments, these single-pixel phototubes can detect, for example, only one “blob” of charge coming from three separate photons in a neutrino-nucleus collision. Now with LAPPDs, scientists can read each individual photon, retrace where the photon came from and determine the time the light was emitted roughly 10 times better than previous photodetectors.
Think of it as tracing photons’ movements at the scale of 50 to 60 trillionths of a second.
High-precision timing with LAPPDs and accurate neutron tagging with the gadolinium-loaded water detector takes neutrino and proton-decay research to another level.
Thirty collaborators, including postdoctoral researchers and students, were very active in building and installing the electronics, water systems and photodetection tubes.
“We’ve done an excellent job of working together and making it happen as quickly as possible,” said Mayly Sanchez, a co-spokesperson for the ANNIE project and an Intensity Frontier fellow at Fermilab.
Now that the ANNIE detector is in its home in the Fermilab SciBooNE building and taking data, the collaboration is preparing to analyze their results.
“For phase one, we will be doing some neutrino background measurements for the ultimate physics measurements that we want to do,” Sanchez said. “The physics measurement in phase two will have an impact both in our knowledge of neutrino interactions and as the first application of a new photodetector technology in high-energy physics.”
Phase one, supported by Fermilab, will continue until the Fermilab accelerator shutdown begins in July. The main physics experimentation and R&D studies will take place during phase two, which awaits funding. ANNIE researchers, supported by the U.S. Department of Energy Office of Science and the National Science Foundation, will later compare results from both phases to see what the experiment yields.
“We learned what it takes to get an experiment like ANNIE off the ground at Fermilab,” Wetstein said. “I’m really proud that we got the whole thing from basically a big proposal to a fully designed experiment to turning all of our systems on within a year.”
ANNIE’s collaborating institutions are Argonne National Laboratory, Fermilab, Iowa State University, Ohio State University, Queen Mary University of London, University of California, Berkeley, University of California, Davis, University of California, Irvine, University of Chicago and University of Sheffield.