Para una versión en español, haga clic aquí. Para a versão em português, clique aqui.
Charged particles, like protons and electrons, can be characterized by the trails of atoms these particles ionize. In contrast, neutrinos and their antiparticle partners almost never ionize atoms, so their interactions have to be pieced together by how they break nuclei apart.
But when the breakup produces a neutron, it can silently carry away a critical piece of information: some of the antineutrino’s energy.
Fermilab’s MINERvA collaboration recently published a paper to quantify the neutrons produced by antineutrinos interacting on a plastic target.
The way antineutrinos change between their various types could help explain why the modern universe is dominated by matter. The most promising model of how this behavior relates particles and antiparticles depends on antineutrino energy. However, neutrons can leave holes in the puzzle of an antineutrino’s identity because they carry away energy and are produced in different quantities by neutrinos and antineutrinos. This MINERvA result is aimed at improving predictions of how neutrons could affect current and future neutrino experiments, including the international Deep Underground Neutrino Experiment, hosted by Fermilab.
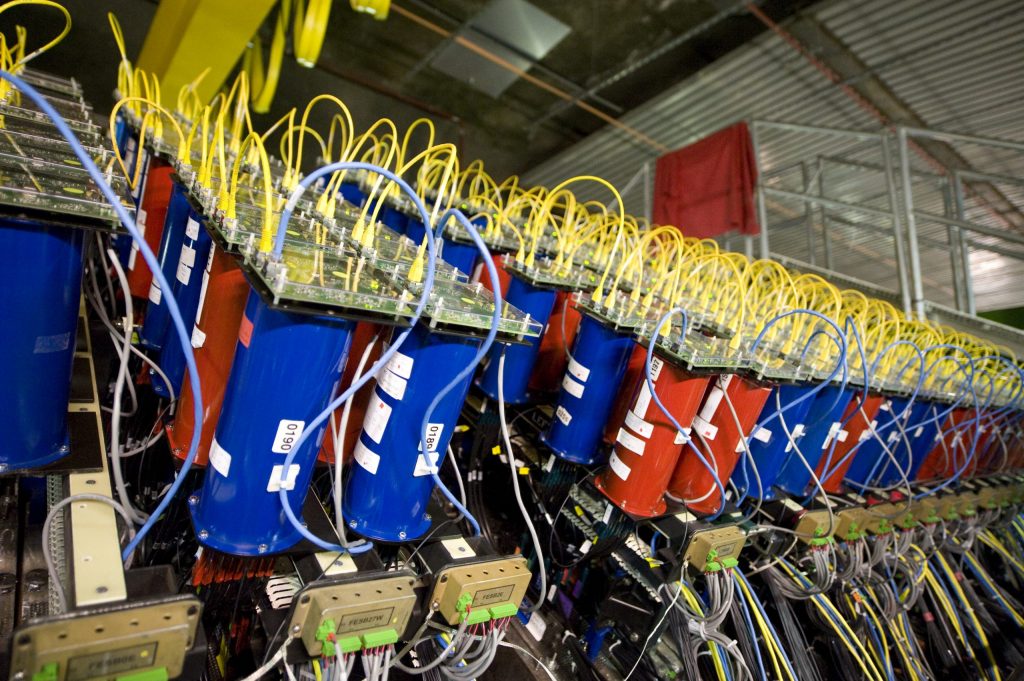
The MINERvA detector at Fermilab helps scientists analyze neutrino interactions with atomic nuclei. Photo: Reidar Hahn
In this study, MINERvA looked for antineutrino interactions that produce neutrons. The antineutrino interactions that MINERvA studies look like one or more trails of ionized atoms all pointing back to a single nucleus. Unlike charged particles, neutrons can travel many tens of centimeters from an antineutrino interaction before being detected. So, the MINERvA collaboration characterized neutron activity as pockets of ionized atoms spatially isolated from both charged particle tracks and the interaction point.
An antineutrino interaction can produce other types of neutral particles, which can fake a neutron interaction, and charged particles, which can confuse a neutron counting measurement by themselves ejecting neutrons from nuclei. In addition, when these charged particles have low momentum, they can end up in a mass of ionization too close to the interaction point to be counted separately that also masks evidence for neutral particles. So, neutrons can be counted more accurately in antineutrino interactions that produce few additional particles. MINERvA scientists used conservation of momentum calculations to avoid interactions that produced many charged particles.
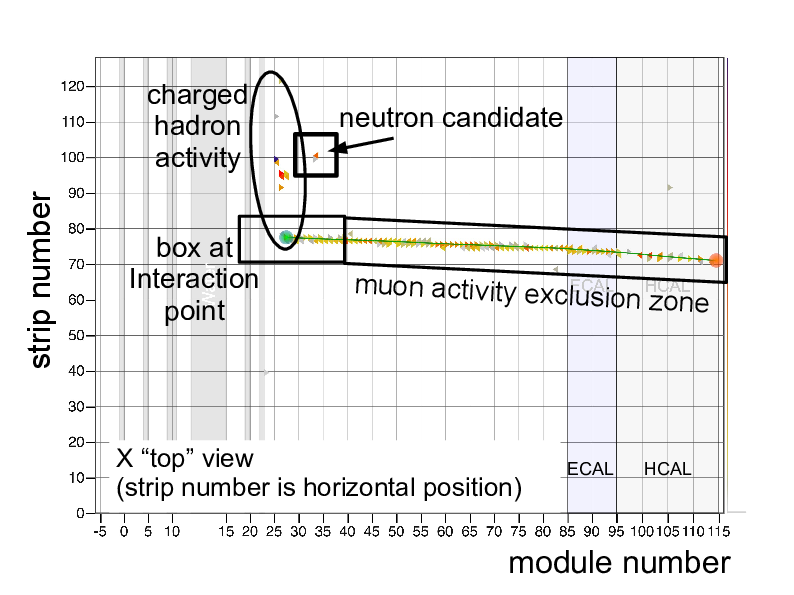
This graphic illustrates a neutrino interaction in the MINERvA detector. The rectangular box highlights the spot where a neutrino interacted inside the detector. The square box just above it highlights the appearance of a neutron resulting from the neutrino interaction. Image: MINERvA
Other experiments’ measurements of neutrons from antineutrinos have waited for each neutron to lose most of its energy before it can be counted. However, neutrons from MINERvA’s antineutrino sample have enough energy to knock other neutrons out of nuclei they collide with. This chain reaction changes both the original neutrons’ energies and the number of neutrons detected. This result focuses on signs of neutrons within tens of nanoseconds of an antineutrino interaction.
By understanding neutron production in concert with MINERvA’s characterization of antineutrino interactions on many nuclei, future oscillation studies can quantify how undetected neutrons could affect their conclusions about the differences between neutrinos and antineutrinos.
Andrew Olivier is a physicist at the University of Rochester and member of the MINERvA collaboration.