Editor’s note: This press release by the Dark Energy Survey collaboration was originally published by the Institut de Física d’Altes Energies about the constraints on dark energy set by the measurement of baryonic acoustic oscillations.
DES is an international collaboration comprising more than 400 astrophysicists, astronomers and cosmologists from over 25 institutions led by members from the U.S. Department of Energy’s Fermi National Accelerator Laboratory. DES mapped an area almost one-eighth of the entire sky using the Dark Energy Camera, a 570-megapixel digital camera funded by the DOE Office of Science. The result highlights research made possible thanks to the unique observing capabilities of the Dark Energy Camera, which was built and tested at Fermilab for the Dark Energy Survey.
“With the Dark Energy Survey, we can peer back in time and measure the universe’s growth with unprecedented accuracy. We are reaching farther into the past than other galaxy surveys,” said DES director and spokesperson Rich Kron, who has worked on the survey as a Fermilab and University of Chicago scientist for more than 10 years. “This latest measurement allowed us to exploit sound waves created about 400,000 years after the Big Bang. Our result has an impressive accuracy of two percent and supports the standard model of the accelerated expansion of the universe.”
- The measurement obtained fixes the scale of the universe when it was half its present age with an accuracy of 2%, the most accurate ever obtained for that time. The results were released yesterday.
- Researchers from the Centro de Investigaciones Energéticas, Medioambientales y Tecnológicas (CIEMAT), the Institut de Ciències de l’Espai (ICE-CSIC,IEEC), the Institut de Física d’Altes Energies (IFAE) and the Instituto de Física Teórica (UAM-CSIC) have led the scientific analysis of the data.
We now have a standard model of cosmology, the current version of the Big Bang theory. Although it has proved very successful, its consequences are staggering. We know only 5% of the content of the universe, which is normal matter. The remaining 95% is made up of two exotic entities that have never been produced in the laboratory and whose physical nature is still unknown. These are dark matter, which accounts for 25% of the content of the cosmos, and dark energy, which contributes 70%. In the standard model of cosmology, dark energy is the energy of empty space, and its density remains constant throughout the evolution of the universe.
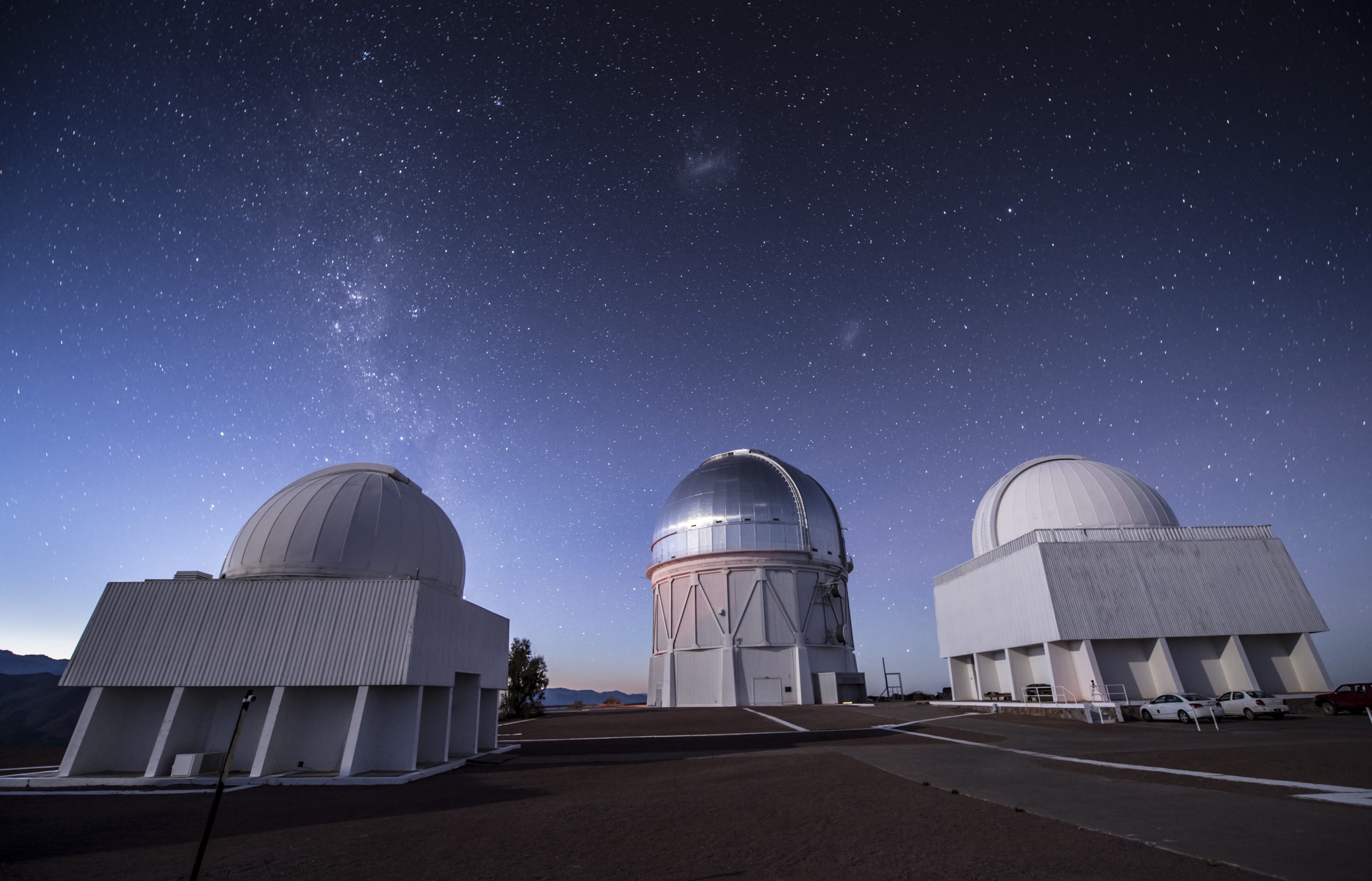
The Cerro Tololo Interamerican Observatory (CTIO) in the Chilean Andes. Image credit: DOE/FNAL/DECam/R. Hahn/CTIO/NOIRLab/NSF/ AURA
According to this theory, sound waves propagated in the very early universe. In those early stages, the universe had an enormous temperature and density. The pressure in this initial gas tried to push the particles that formed it apart, while gravity tried to pull them together, and the competition between the two forces created sound waves that propagated from the beginning of the universe until about 400,000 years after the Big Bang. At that time, the radiation and matter stopped interacting, and the waves were frozen, leaving an imprint on the spatial distribution of matter. This imprint is observed as a small preferential accumulation of galaxies separated by a characteristic distance, called the Baryon Acoustic Oscillations (BAO) scale by cosmologists, and corresponds to the distance traveled by the sound waves in those 400,000 years.
A new measurement of the cosmic distance
The Dark Energy Survey (DES) has just measured the BAO scale when the universe was half its present age with an accuracy of 2%, the most accurate determination yet at such an early epoch, and the first time an imaging-only measurement is competitive with large spectroscopy campaigns specifically designed to detect this signal.
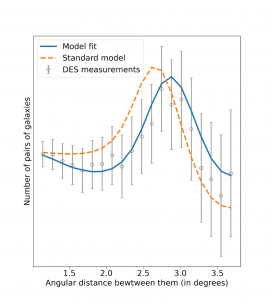
Signal from Baryon Acoustic Oscillations (BAO) in the Dark Energy Survey (DES) data. When plotting the number of galaxy pairs as a function of their angular separation in the sky, we find an excess of pairs at 2.90 degrees. This is caused by BAO waves that have traveled hundreds of millions of light-years since the Big Bang. These waves subtend a size on the sky somewhat larger than predicted by the standard model of cosmology and the Planck data. Image credit: Dark Energy Survey Collaboration.
The distance the sound wave travels in the early universe depends on well-known physical processes, so it can be determined with great precision, setting a yardstick for the universe. It is what cosmologists call a standard ruler. In this case, it has a length of about 500 million light-years. By observing the angle that this standard ruler subtends in the sky at different distances (or, in other words, at different epochs in the universe), one can determine the history of the cosmic expansion and, with it, the physical properties of dark energy. In particular, it can be determined by analyzing the cosmic microwave background, the radiation released when atoms were formed, 400,000 years after the Big Bang that gives us a snapshot of the very early universe, as published by the Planck collaboration in 2018. It can also be determined in the late universe by studying the BAO scale in galaxy mappings as DES has done. Analyzing the consistency of both determinations is one of the most demanding tests of the standard model of cosmology.
“It is a source of pride to see how, after almost twenty years of continuous effort, DES produces scientific results of the highest relevance in cosmology,” says Eusebio Sánchez, head of the cosmology group at CIEMAT. “It is an excellent reward for the effort invested in the project.”
“What we observed is that galaxies have a greater tendency to be separated from each other by an angle of 2.90 degrees on the sky compared to other distances,” comments Santiago Ávila, a postdoctoral researcher at IFAE and one of the coordinators of the analysis. “That’s the signal! The wave can be seen clearly in the data,” he adds, referring to the first figure. “It’s a subtle preference, but statistically relevant,” he says, “and we can determine the wave pattern with an accuracy of 2%. For reference, the full moon occupies half a degree in diameter in the sky. So if we were able to see the galaxies with the naked eye, the BAO distance would look like 6 full moons.”
16 million galaxies to measure the universe 7 billion years ago
To measure the BAO scale, DES has used 16 million galaxies, distributed over one-eighth of the sky, that have been specially selected to determine how far away they are with sufficient precision.
“It is important to select a sample of galaxies that allows us to measure the BAO scale as accurately as possible,” says Juan Mena, who did his Ph.D. at CIEMAT on this study and is now a postdoctoral researcher at the Laboratory of Subatomic Physics and Cosmology in Grenoble (France). “Our sample is optimized to have a good compromise between a larger number of galaxies and the certainty with which we can determine their distance.”
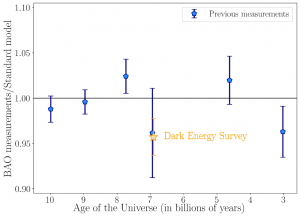
In gold, we see the Dark Energy Survey BAO scale measurement, which deviates from the standard model (horizontal line at 1 in this plot) by 4%, while the uncertainties associated with the analysis are 2% (indicated by the golden vertical bar). This discrepancy could be a clue about dark energy or a mere statistical fluctuation, with a 5% chance. This measurement has been made by observing galaxies that emitted their light when the Universe, which is 14 billion years old, was about half its present age. In blue are shown measurements from the Baryonic Oscillations Spectroscopic Survey (BOSS) and its extension (eBOSS). DES gives us the most accurate measurement of when the Universe was about 7 billion years old. Image credit: Dark Energy Survey Collaboration.
Cosmological distances are so large that light takes billions of years to reach us, thus allowing us to observe the cosmic past. The sample of galaxies used in this study opens a window into the universe seven billion years ago, slightly less than half its present age.
“One of the most complicated tasks in the process is to clean the galaxy sample of observational contaminants: distinguishing between galaxies and stars or mitigating the effects of the atmosphere on the images,” says Martín Rodríguez Monroy, a postdoctoral researcher at the IFT in Madrid.
Clues about the mysterious dark energy
An interesting finding of this study is that the size these waves occupy on the sky is 4% larger than predicted from measurements made by ESA’s Planck satellite in the early universe using the cosmic microwave background radiation. Given the sample of galaxies and the uncertainties of the analysis, this discrepancy has a 5% chance of being a mere statistical fluctuation. If it were not, we could be looking at one of the first clues that the current theory of cosmology is not quite complete, and the physical nature of the dark components is even more exotic than previously thought. “For example, dark energy may not be the energy of the vacuum. Its density may change with the expansion of the universe, or even space may be slightly curved,” says Anna Porredon, a Spanish researcher at the Ruhr University Bochum (RUB) in Germany. This researcher, a fellow of the Marie Skłodowska-Curie Actions program of the European Union, has been one of the coordinators of this analysis.
The BAO scale has been measured by other cosmological projects before DES at different ages of the universe, mainly the Baryonic Oscillation Spectroscopic Survey (BOSS) and its extension (eBOSS), which were designed for this purpose (see second figure). However, the DES measurement is the most accurate at such an early age of the universe, with half the uncertainty of eBOSS at that time. The significant increase in precision has made it possible to reveal the possible discrepancy in the BAO scale with respect to the standard model of cosmology.
“To follow this lead, the next crucial step is to combine this information with other techniques explored by DES to understand the nature of dark energy”, comments Hugo Camacho, a postdoctoral researcher at Brookhaven National Laboratory (USA), formerly at the Institute of Theoretical Physics at São Paulo State University in Brazil (IFT-UNESP) and member of the Laboratorio Interinstitucional de e-Astronomia (LIneA), and adds “Moreover, DES also paves the way for a new era of discoveries in cosmology, which will be followed by future experiments with even more precise measurements.”
The Dark Energy Survey
As its name suggests, DES is a large cosmological project specially conceived to study the properties of dark energy. It is an international collaboration of more than 400 scientists from seven countries with its headquarters at the U.S. Department of Energy’s Fermi National Accelerator Laboratory, near Chicago. The project is designed to use four mutually complementary methods: cosmological distances with supernovae, the number of galaxy clusters, the spatial distribution of galaxies, and the weak gravitational lensing effect (more details at https://www.darkenergysurvey.org/the-des-project/science/).
In addition, these methods can be combined to obtain higher statistical power and better control of the observations, which are expected to be consistent. The combination of the gravitational lensing effect with the spatial distribution of galaxies is especially relevant. These analyses test the cosmological model in a very demanding way. Results using half of the DES data have already been published to great acclaim, and the final measurements, using the full data set of more than 150 million galaxies, are expected to be published later this year. “DES allows us to understand for the first time whether the accelerating expansion of the Universe, which began 6 billion years ago, is consistent with our current model for the origin of the Universe,” comments Martin Crocce, who co-coordinates this latest analysis from ICE.
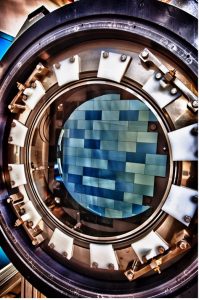
Focal plane of DECam (Dark Energy Camera). It contains 62 ultra-sensitive CCD sensors specially designed for the DES project, and allows imaging of the universe with unprecedented depth. Image credit: DOE/FNAL/DECam/R. Hahn/CTIO/NOIRLab/NSF/ AURA
To use all these techniques, the DES built the 570 Megapixel Dark Energy Camera (DECam), one of the largest and most sensitive cameras in the world. It is installed on the Víctor M. Blanco telescope, with a 4m diameter mirror, at the Cerro Tololo Inter-American Observatory in Chile, operated by the US NSF’s NOIRLab. DES has mapped one-eighth of the celestial vault to an unprecedented depth. It took 4-color images between 2013 and 2019 and is currently in the final phase of the scientific analysis of these images. Spanish institutions have been part of the project since its inception in 2005 and, in addition to having collaborated prominently in the design, manufacture, testing, and installation of DECam and data acquisition, have important responsibilities in DES scientific management to date.
More information is available at www.darkenergysurvey.org/collaboration.
DES contacts:
IFAE – Dr. Santiago Ávila, postdoctoral researcher, savila@ifae.es
CIEMAT – Dr. Eusebio Sánchez, research scientist, eusebio.sanchez@ciemat.es
IFT-UAM/CSIC – Dr. Martín Rodríguez-Monroy, postdoctoral researcher, martin.rodriguez@uam.es
ICE – Dr. Martín Crocce, tenured scientist, crocce@ice.csic.es
Reference: https://arxiv.org/abs/2402.10696
Fermi National Accelerator Laboratory is America’s premier national laboratory for particle physics research. A U.S. Department of Energy Office of Science laboratory, Fermilab is located near Chicago, Illinois, and operated under contract by the Fermi Research Alliance LLC. Visit Fermilab’s website at https://www.fnal.gov and follow us on Twitter @Fermilab.
The DOE Office of Science is the single largest supporter of basic research in the physical sciences in the United States and is working to address some of the most pressing challenges of our time. For more information, please visit science.energy.gov.