At present scientists think that at the highest energies and earliest moments in time, all the fundamental forces may have existed as a single unified force. As the universe cooled just one microsecond after the Big Bang, it underwent a “phase transition” that transformed or “broke” the unified electromagnetic and weak forces into the distinct forces observed today.
One can compare the phase transition to the transformation of water into ice. In this familiar case, we call the transition a change in a state of matter. In the early-universe case, we call the transition electroweak symmetry breaking.
In the same way that we characterize the water-to-ice phase transition as occurring when the temperature drops below 32 degrees, we characterize the amount of symmetry breaking with a parameter called the weak mixing angle, whose value has been measured over the years.
By recreating the early-universe conditions in accelerator experiments, we have observed this transition and can measure the weak mixing angle that controls it. Our best understanding of the electroweak symmetry breaking involves the so-called Higgs mechanism, and the Nobel Prize-winning Higgs boson discovery in 2012 was a milestone in our understanding.
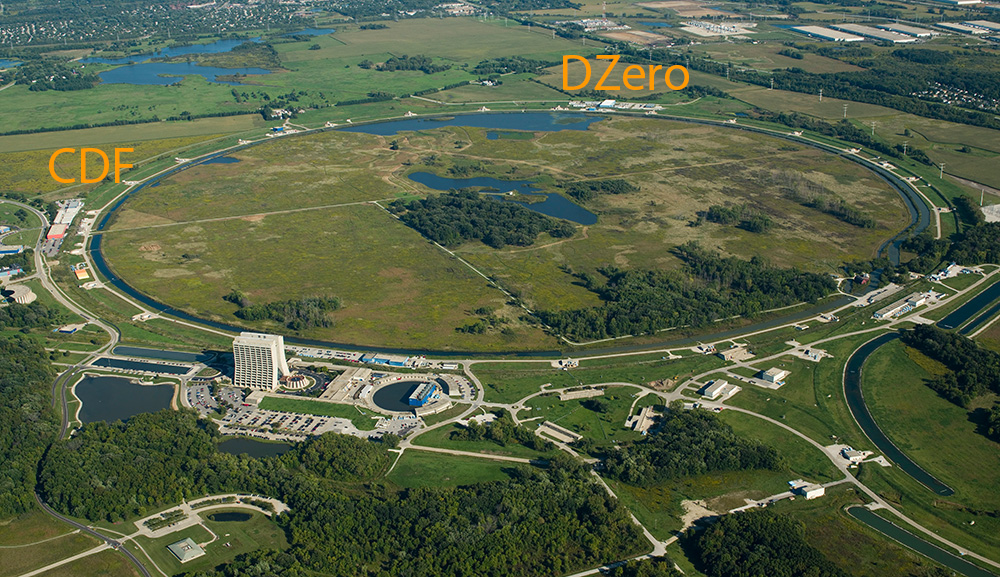
The CDF and DZero experiments at the Tevatron have published their latest measurement of the electroweak mixing angle. Photo: Reidar Hahn
Measuring the weak mixing angle: a global pursuit
Previous determinations of the weak mixing angle from around the world disagreed, allowing for the possibility that maybe there are new fundamental particles to be discovered, or maybe even pointing to a misunderstanding in how we think about the fundamental forces. A new result from Fermilab helps to resolve the discrepancy and reinforces our standard theory of the fundamental forces.
Our combined understanding of particle physics and cosmology hinges on our understanding of the particles and their interactions: how the Higgs boson breaks the symmetry, how the different forces mesh with each other, whether there are new and unknown particles like dark matter or other unseen forces that could have a dramatic effect on the evolution of the universe.
The details of this symmetry breaking affect why matter is stable at all and how stars and galaxies form. The much improved agreement on the measurement of the weak mixing angle helps cement our understanding of the past, the character of what we observe today, and what we believe is in store for our future.
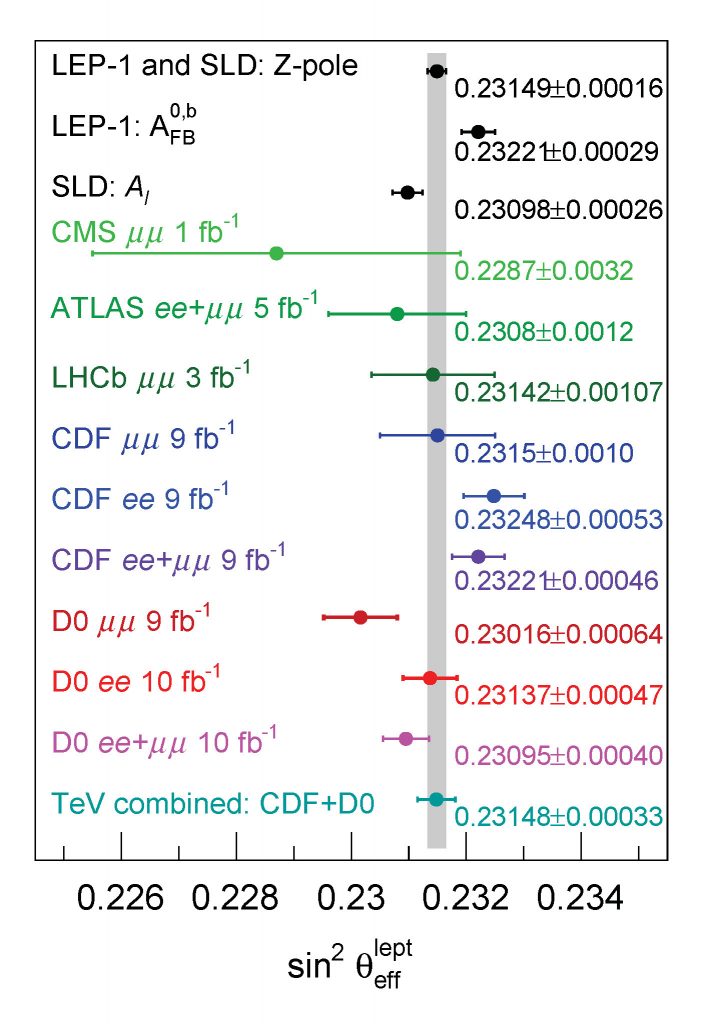
This is a compilation of measurements of the weak mixing angle. The new Tevatron combined result of sin2θefflept=0.23148 ± 0.00033 rivals the precision of the two previous measurements by the LEP-1 experiments at CERN and SLD at SLAC, and it lies midway between them. The Tevatron combination agrees very well with the world average of all results shown by the shaded band.
For two decades, the most precise measurements of the weak mixing angle came from experiments that collided electrons and positrons at the European laboratory CERN and SLAC National Accelerator Laboratory in California, each of which gave different answers. Their results have been puzzling because the probability of the two measurements to agree was less than one part in a thousand, suggesting the possibility of new phenomena. More input was needed.
Although the environment in Fermilab’s proton-antiproton Tevatron Collider was much harsher than either CERN’s or SLAC’s collider, with many more background particles, the large and well-understood data sets of the Tevatron’s CDF and DZero experiments allowed a new combined measurement that gives almost the same precision as the electron-positron measurements.
The new measurement of the mixing angle sin2θefflept is 0.23148 ± 0.00033, and it lies about midway between the CERN and SLAC measurements and thus is in good agreement with both of them, as well as with the average of all previous direct and indirect measurements of weak mixing. Thus, Occam’s razor suggests that those new particles and forces are not yet necessary and that our present particle physics and cosmology models remain good descriptors of the observed universe.
Read more in Physical Review D and on the Tevatron Run II webpage.
Breese Quinn is a member of the DZero collaboration and a physicist at the University of Mississippi. Willis Sakumoto is a member of the CDF collaboration and a physicist at the University of Rochester.