The latest results from the Fermilab NOvA experiment are taking us closer to describing the most basic properties of the mysterious neutrino — the most abundant particle of matter in the universe.
Neutrinos appear in a variety of natural processes, from formidable supernova explosions and nuclear reactions in the sun to radioactive decays in your banana. They are also produced in abundance in nuclear reactors and particle accelerators. Yet neutrinos barely interact with matter: a light-year of lead would hardly stop your average neutrino. Their elusive nature makes them extremely challenging to study, which explains both why we still know very little about their properties and why many scientists and experiments around the world have so much fun hunting them down.
The observation that neutrinos are able to change type — a behavior called oscillation — proved that neutrinos have masses, albeit very small. This phenomenon explains how neutrinos that are produced in one of the three “flavor” states (electron neutrino, muon neutrino or tau neutrino) transition in and out of these types as they travel a certain distance and may be detected as a different type. The probability of these transitions depends on a number of factors: the energy of the neutrino, the distance between the particle beam source and the detector, the differences in neutrino masses, the amount of blending between neutrino types, which scientists describe with three “mixing” angles, and additional complex phases.
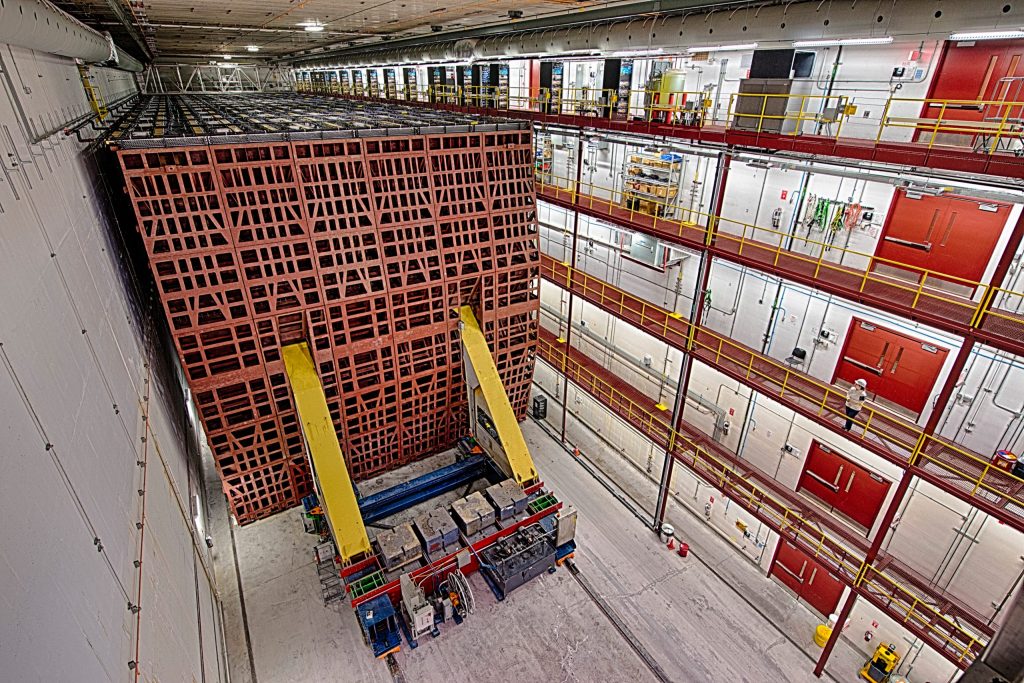
Fermilab’s NOvA neutrino experiment studies neutrino oscillations using a powerful neutrino beam produced by the lab’s accelerator complex. The beam, made of muon neutrinos, is sent to NOvA’s two detectors — one located at Fermilab and one located about 800 kilometers away in Minnesota, pictured here.
Fermilab’s NOvA neutrino experiment studies neutrino oscillations using the powerful NuMI neutrino beam produced by the lab’s accelerator complex. The beam, made of muon neutrinos, is sent to NOvA’s two detectors — one located at Fermilab and one located about 800 kilometers away in Minnesota. The NOvA far detector looks to identify the fraction of muon neutrinos in the NuMI beam that oscillated into electron neutrinos (called electron neutrino appearance) and the fraction of muon neutrinos that oscillated to a different flavor (called muon neutrino disappearance).
The NuMI beam is generally described as a muon neutrino beam, but it can also be made of muon antineutrinos. The antineutrino is the antiparticle of the neutrino. Just as muon neutrinos can oscillate into electron neutrinos, muon antineutrinos can oscillate into electron antineutrinos.
Experimentalists can use information from the combination of the measurements of electron and muon neutrinos, as well as their antiparticle equivalents, to draw their conclusions. For example, if the oscillation rates of antineutrinos compared to those of neutrinos are different, the implication could be a violation of a symmetry called charge parity, commonly called CP. The existence of this type of CP violation is one of the great unknowns in particle physics that NOvA is investigating.
NOvA’s latest measurements of neutrino oscillation parameters have been published in Physical Review Letters. The data were recorded between 2014 and 2019 and correspond to 8.85 x 1020 protons-on-target of neutrino beam and 12.33 x 1020 protons-on-target of antineutrino beam. This represents a 78% increase in the amount of antineutrino data compared to NOvA’s previous results, presented at the Neutrino 2018 conference.
NOvA identified 27 electron antineutrino candidate events in the NOvA far detector, compared to the 10.3 events expected if muon antineutrinos did not oscillate into electron antineutrinos. This remains the strongest evidence (4.4 sigma) of electron antineutrino appearance in a muon antineutrino beam for a long-baseline experiment. (In particle physics, 3 sigma is usually considered “strong evidence” that the conclusions of the data analysis are unlikely to be a fluke, while 5 sigma means that the experimental results qualify as a discovery.)
In addition to those 27 electron antineutrino events, 102 surviving muon antineutrino candidates were detected in the far detector, where 476 events would have been expected if muon antineutrinos did not oscillate at all. NOvA scientists combined these new events with previously recorded neutrino data and analyzed them jointly. Pictures of such neutrino and antineutrino events as recorded by the NOvA far detector are shown below.
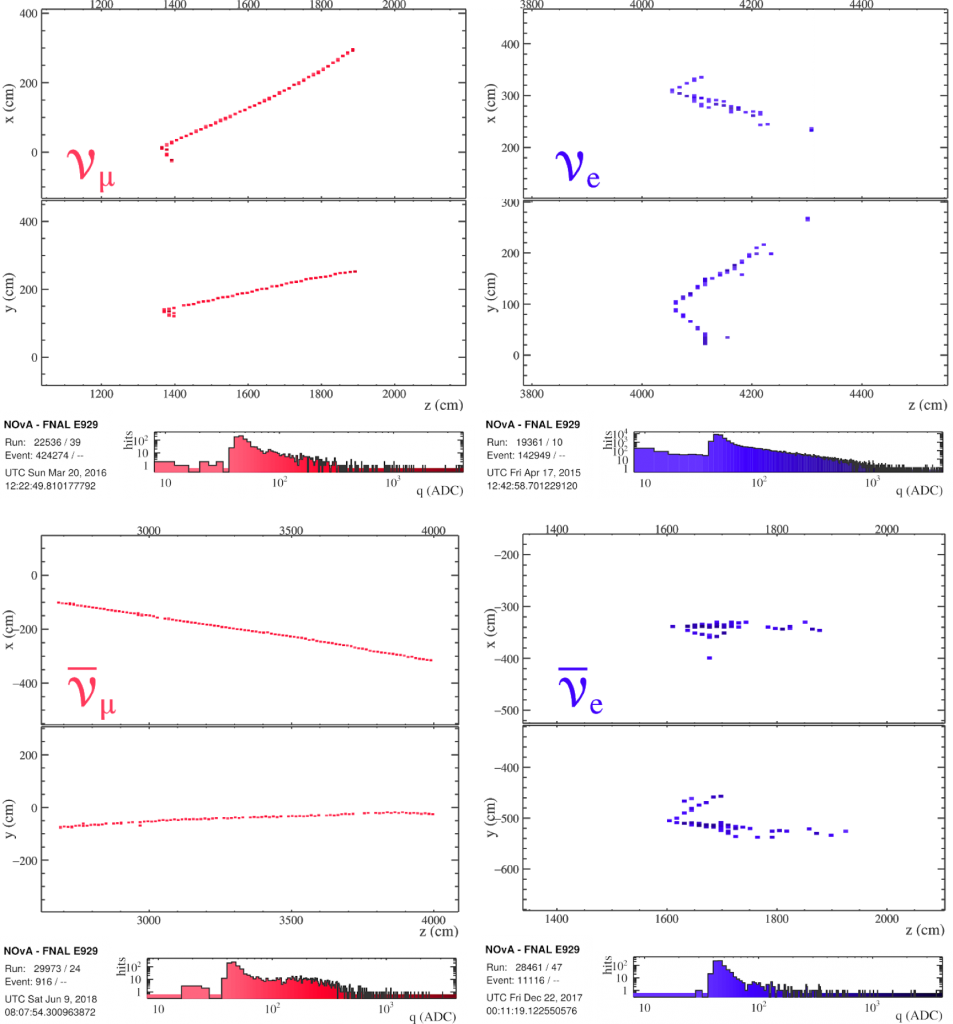
Four events observed in the NOvA far detector, classified as muon (left) or electron (right) neutrino interactions, with the beam in neutrino (top) or antineutrino (bottom) mode. Each panel shows two views of the same event, and the color represents the energy deposited by particles that emerged from the interaction. The latest NOvA results comprise four data samples with 113 muon neutrino to muon neutrino, 58 muon neutrino to electron neutrino, 102 muon antineutrino to muon antineutrino and 27 muon antineutrino to electron antineutrino candidates.
The results help scientists chip away another problem in neutrino physics: the ordering of the three neutrino masses — which of the three is the lightest? NOvA’s combined neutrino-antineutrino appearance and disappearance fit shows a preference (1.9 sigma) for what is called normal mass ordering: The three neutrino mass states are ordered m1 ≤ m2 ≤ m3.
NOvA is also working to measure one of the least known oscillation parameters, θ23, that governs the degree of flavor mixing in the third mass state. The fit shows a slight preference (1.6 sigma) for the value of this angle to be in the upper octant (θ23 > 45 degrees) and therefore points towards an absence of symmetry in the way muon and tau neutrino flavors contribute to the third neutrino mass state. The data recorded thus far does not allow us to draw conclusions about CP violation in neutrino interactions.
The experiment is scheduled to collect new data until 2025. NOvA collaborators are continually working to improve the experiment and analysis techniques to potentially provide a definitive statement about the neutrino mass ordering, the value of θ23, and strong constraints on the CP-violating phase. These measurements are paramount if we want to understand the neutrino properties and the role they played in the formation of the universe as we know it.
This work is supported in part by the DOE Office of Science and the National Science Foundation.
Steven Calvez is a Colorado State University scientist on the NOvA experiment. Erika Catano Mur is a William & Mary scientist on the NOvA experiment.