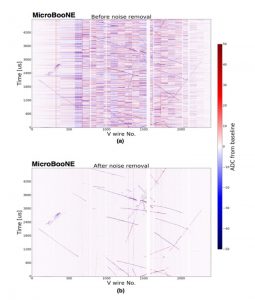
This two-dimensional event display shows the raw signal (a) before and (b) after offline noise filtering. Clean event signatures were recovered once all excess noise was removed.
If you have ever tried to watch a movie or listen to music on a plane, then you know the problem well: The roar of the engines makes it difficult to hear what’s being piped through the speakers — even when those speakers are situated in or on your ear. It’s great that we have noise-canceling headphones that can provide a more enjoyable listening experience. High-frequency sound waves are filtered because of the very structure of the headphone. Low-frequency sound waves are filtered by a process called destructive interference: The headphone’s sound waves are created 180 degrees out of phase with the intruding waves — those of the airplane engines — and the two waves cancel each other out. As a result, the listener can focus on the sounds he or she wants to hear.
In a similar manner, at the MicroBooNE detector we identify and filter out several excess noise sources at both high and lower frequencies. The primary component of the MicroBooNE detector is the liquid-argon time projection chamber (LArTPC) — a large, horizontal-silo-like vessel filled with liquid argon. Scientists make measurements of the particles that result from the collision of a neutrino with an argon nucleus. These particles leave in the argon a trail of electrons, which drift toward two planes of wires —8,256 of them — in the LArTPC. That information travels as current through the wires, and researchers use the information to learn more about the neutrino that triggered it.
The current on each wire is amplified and shaped by custom low-power, low-noise circuits immersed in the liquid argon. The low-noise operation of readout electronics in a LArTPC is critical to properly extract the distribution of charge deposited on the wire planes of the TPC. Using data from the first year of MicroBooNE operations, researchers identified several distinct types of TPC noise, which exceeded expectations for the types of noise inherent to the electronics.
With the support from the entire collaboration, the analysis team developed an offline noise filter that eliminates most of the excess noise while achieving excellent signal preservation. The resulting two-dimensional event display for a signal from one data event is shown in the figure both before (panel (a)) and after (panel (b)) offline noise filtering. The team recovered clean event signatures after subtracting all identified noise sources. The residual electronics noise is consistent with the cold electronics design expectations and is stable with time and uniform over the functioning channels. This noise level is significantly lower than previous experiments that used warm front-end electronics.
In summer of 2016, the collaboration additionally performed several hardware upgrades, with new components from Brookhaven National Laboratory and technical support from Fermilab, to mitigate the two largest sources of excess noise. After the successful upgrade, the residual excess noise was largely removed. This result has been submitted to the Journal of Instrumentation and can be found on arXiv.
In a talk on the topic, Jyoti Joshi of Brookhaven, one of the papers co-authors, noted that the experience accumulated during the first year of data taking proved to be critical in the optimization and operation of the MicroBooNE TPC and is already proving useful in informing the design of future LArTPC detectors. There will be a wine and cheese seminar at Fermilab covering the first year of MicroBooNE operations on July 28, so come learn all about it.